Volume 11 - Year 2023 - Pages 01-09
DOI: 10.11159/ijepr.2023.001
Restoration of Heterogeneous Soils Contaminated with Copper Using Electrokinetic Remediation
Ikrema Hassan 1, Eltayeb Mohamedelhassan2
1
University of New Brunswick, Department of Engineering, Saint John
Ikrema.hassan@unb.ca
100 Tucker Park Rd, Saint John, New Brunswick, Canada
2Lakehead University, Department of Civil Engineering
966 Oliver Rd, Thunder Bay, Ontario, Canada
Eltayeb@lakeheadu.ca
Abstract - In this study, heterogeneous soils contaminated with copper were remediated using solar powered electrokinetic treatment. The heterogeneous soils were composed of clay and sand with ratio 2:1. In one soil, a sand layer was sandwiched between two layers of clay while in another sand pockets made 1/3 of the soil mass. The third heterogeneous soil was a clay-sand mixture. An additional test was carried out with homogeneous clay to provide data for contrast. The soil samples were artificially contaminated with 150 mg of copper per kg of dry soil at water content 41% and placed inside four identical electrokinetic cells. Each cell was connected to a solar cell panel with peak voltage gradient 205 V/m. Encouraging results were obtained. Eighty-seven percent of copper was removed from specimen near the anode in the test of the clay-sand mixture compared to 86% in the homogeneous clay.
Keywords: Electrochemical, Heterogeneous, Contamination, Heavy metals, Clay, Copper.
© Copyright 2023 Authors - This is an Open Access article published under the Creative Commons Attribution License terms. Unrestricted use, distribution, and reproduction in any medium are permitted, provided the original work is properly cited.
Date Received: 2023-04-02
Date Revised: 2023-05-10
Date Accepted: 2023-05-21
Date Published: 2023-06-26
1. Introduction
Improper management of mine tailings and industrial waste pose major threats to the environment around the globe. Mine tailings usually contain a substantial amount of heavy metals which can cause soil contamination and consequently ground water pollution [1] [2]. Electrokinetic remediation technique is a great promise for remediation of contaminated soils as it has high removal efficiency and time effectiveness [3]. The main advantages of electrokinetic remediation treatment over conventional soil treatment methods such as thermal desorption, soil vapour extraction, and soil washing are that it can be performed in situ and it is effective in cleaning fine-grained soils (which are the most difficult to remediate) with low hydraulic permeability [4]. According to Buchireddy et al. (2009), among many factors, the contaminated soil characteristic either homogeneous or heterogeneous plays a dominant role in the selection of suitable remediation technology [5]. Due to the wide varieties in contaminated site conditions, environmental scientists believe that there will not be a single method suitable for all different situations [5]. Rather implementation of integrated technologies can be efficient and cost effective. Despite the numerous studies, the remedy of heterogeneous soils by conventional methods remains to be a great challenge. For instance, in situ soil flushing process is ineffective in heterogeneous soils because of the fact that the flushing fluid tends to flow through the soil pore in paths of high permeability [6]. This normally results in removal of the contaminant from the soil part with high permeability by flushing effect, whereas for the soil part with low permeability only the limited diffusion mechanism will control the remediation process. On the other hand, electrokinetic remediation processes have a great control over the electric field distribution on the soil under treatment. The orientation of the electrodes directs the electrical current and consequently controls the contaminant transport in soil strata [7]. This capability can makes electrokinetic remediation a better alternative for remediation of heterogeneous soils. Although, most of the electorkinetic remediation investigations were done on homogeneous soils, limited tests have been done to study heterogeneous soils [8].
2. Related Work
Electrokinetic remediation is an emerging technology for cleaning up contaminated soils. It can be particularly effective in fine-grained soils, which are difficult to be clean by common flushing method, as the technology has the ability to promote fluid and mass migration through soil with low permeability [2-3]. The contaminants are mobilized and eventually recovered by passing a low-level direct current (dc) between a row of positively charge electrodes (anode) and negatively charged electrodes (cathode) inserted into the ground [2-3]. Electrokinetic exploits two transport mechanisms: electroosmosis and electromigration. Electroosmosis is the movement of water in the soil pores from the anode to cathode that results from an applied electric field gradient and electromigration is the movement of ions in the soil pore fluid towards the oppositely charged electrodes [9]. Electrolysis reactions occur at the electrodes in an electrokinetic process. The reactions results in oxidation at the anode generating an acid front and reduction at the cathode producing a base front.
Over the last decade, solar energy has globally attracted increased interests among researchers and engineers. The fact that solar cell generates direct current (DC), not alternating current (AC), make the solar cells an excellent candidate to generate the power supply required for electrokinetic remediation. On the top of that, the power produce by solar cell panel is environmentally friendly and can be suitable for conducting electrokinetic remediation for contaminated soils located in remote areas where there are no or insufficient power supply lines. Moreover, the use of solar cell panel as a source of power can cut short the electricity transmission expenses, power losses in transmission lines, and the use of electrical equipments such as DC transformers. Also, the expected reduction in solar cell prices by time as the technology improved can reduce the initial cost of solar system. The power generated by solar cell panel depends solely on the climate conditions of the treatment location area. This can cause fluctuation in the power supply during the day and intervals of zero power supply at night. In previous studies electrokinetic treatment application, the variations in the supplied power by executing predetermined on and off periods, during the process was found to be beneficial to effectiveness of the treatment [5] [10]. A recent study concluded that in Chain the cost of electorkinetic remediation with solar cell panel was 40% of that carried by electric power from the grid [6] [11].
In this study solar cell panels were used as an alternative power source to generate the electric field required for the electrokinetic remediation for three heterogeneous and one homogenous soils. Copper was used in the experiments to artificially contaminate the soils. The objectives of this study are to investigate the efficiency of the power generated by solar cell panels and to evaluate the influence of soil heterogeneity in electrokinetic remediation processes.
3. EXPERIMENTAL PROGRAM
3.1. Soil Properties
The heterogeneous soils were laboratory-prepared using clay and sand. The homogenous soil consisted of clay. The clay soil was obtained from Plainsman Clay in Medicine Hat, Alberta. X-ray diffraction analysis for the soil revealed that kaolinite is the predominant clay mineral. The Unified Soil Classification System (USCS) group symbol for the clay soil is CL (lean clay). Table 1 summarizes the properties of the clay soil. The sand was sieved from a pile of natural sand of fine to coarse particles. The sand parties passed Sieve No. 30 (0.6 mm) and retained on Sieve No. 40 (0.425 mm) were used to prepare the soil specimens. The sand was washed using Sieve No. 200 (0.075 mm) prior to the test. The USCS group symbol of the sand is SP (poorly graded).
Table 1. Characteristics of clay soils.
Characteristics |
|
Liquid limit |
41 |
Plastic limit |
19 |
Water content (%)1 |
41 |
Sand (%) |
0 |
Silt (%) |
58 |
Clay (%) Specific gravity |
42 2.64 |
Cation Exchange Capacity |
8.9 meq/100 g of soil |
3.2. Experimental Apparatus
The experimental equipment consisted of four identical electrokinetic treatment cells, four solar cell panels, and pore fluid squeezer cell. The electrokinetic cells were designed and fabricated to perform the tests of the study. The general design considerations of the cell were:
- Vertical electrodes configuration. The vertical electrodes layout was selected for its practicality in field installation and the ease of replacing electrode.
- Capability to apply a surcharge load to the soil specimen. The surcharge load can be used to simulate in-situ stress conditions, and to produce soil samples with various void ratios.
The electrokinetic treatment cell, constructed of clear Plexiglas plates 15 mm in thickness, has inner dimensions of 38.5×125×250 mm (length×width×height). The cell is composed of an upper part, a base, and two movable rectangular perforated Plexiglas, 250 mm × 125 mm. The upper part forms outer boundary to accommodate the soil specimen. The rectangular perforated Plexiglas can be used to adjust the soil specimen length between 200 mm and 320 mm. The base of the cell is detachable to allow for easy recovery and minimum disturbance for the soil samples that to be used for subsequent parametric studies. Two drainage valves, used to collect water during the treatment, were installed at the far ends of the base. The voltage across the soil specimen during the test is monitored via four voltage probes installed along the base of the cell. Figure 1 shows the schematic of the electrokinetic treatment cell. Four solar cell panels (NT-175U1) were used as power supply. The solar cell dimension is 1590x820 mm. The open circuit voltage and the maximum current are 41 V and 4.95 A, respectively. Soil pore fluid squeezer cell was especially designed and manufactured to recover pore fluid solution for subsequent testing. It composes of steel cylinder, steel piston, upper casing, porous plate, and lower casing for collecting and discharging the soil pore fluid. The steel cylinder inner dimensions are 50 mm diameter and 100 mm length.
3. 3. Testing Procedure
In this study, copper was the heavy metal used to artificially contaminate the soil. The copper salt was copper (II) chloride dihydrate (CuCl2.2(H2O)). The three heterogeneous soils were clay-sand mixture, clay-sand layers consisting of two layers of clay sandwiching a layer of sand, and clay with sand pockets. The ratio of the clay to the sand was 2:1 (mass/mass) for the three soils. The homogenous soil was clay. The soil was weighted and the volume of water required for a water content of 41% (similar to the liquid limit of the clay) was measured and poured in a container. Copper (II) chloride dihydrate was then added to the container to achieve concentration of 150 mg of copper per kg of dry soil. The soil specimens of the clay-sand mixture and the homogenous clay were prepared by thoroughly mixing the soil with the copper solution using a heavy duty kitchen mixer to water content 41%. The clay and sand layers specimen and the clay with sand pockets specimen were prepared by thoroughly mixing the clay with the copper solution for water content of 52% and mixing the sand with the copper solution for water content of 19%, i.e. the average water content and copper concentration of the soil specimens were 41% and 150 mg per kg of dry soil. Nineteen percent is the maximum water content to be retained by the sand. The contaminated soils were poured in separate heavy duty plastic bags and placed on bails with airtight covers. The soil samples were stored to allow for the copper adsorption from the soil pore fluid to take place and reach equilibrium.
One dimensional electrokinetic remediation was performed in this study. Two perforated graphite electrodes, one served as anode and the other as cathode, of 250×125 ×3 mm (length×width×thickness) were placed 200 mm apart in direct contact with the soil in each of the electrokinetic cells. The electrode covered the entire cross-sectional area of the soil under treatments, and therefore the electric field was uniform across the area (one dimensional electric field). Two geotextile filters were wetted by tap water and placed to cover the other side of the electrode facing the Plexiglas mesh. After 72 hr of preparing the contaminated soil samples, the pre-contaminated soils were placed in each of the four cells in three layers. Each layer was rodded 25 times using steel rod, 16 mm in diameter and 600 mm long with a hemispherically shaped tip, to prevent the entrapment of air pockets. The high water content of the soil and the thorough rodding during placement in the cell insured that the soil specimen was nearly, if not fully, saturated. A surcharge load of 12.8 kg (corresponding to a pressure of 5 kPa) was applied to the soil via the loading plate in four increments over a period of four days. The first surcharge load was 0.6 kg, followed by 3 kg, 5 kg, 7.8 kg, and 12.8 kg.
The longitude and latitude of Thunder Bay city, where the experiments were performed, are 89°14’ W and 48° 24’ N, respectively. During the treatment the solar cell panels were mounted on a wood frame and tilted at an angle of 48° in such way that the bottom of the cell was elevated by 250 mm from the ground. Each solar cell panel was connected to the two graphite electrodes in one of electrokinetic cells. Electric current, voltage across the soil, and water collected in the graduated cylinder (see Figure 1) were monitored and reported during the test. are 11 pt bold Cambria. Similarly, auto-numbering is to be avoided. If more levels of headings are to be used (for example 4. 1. 1.), similar formatting (11 pt bold Cambria) applies.
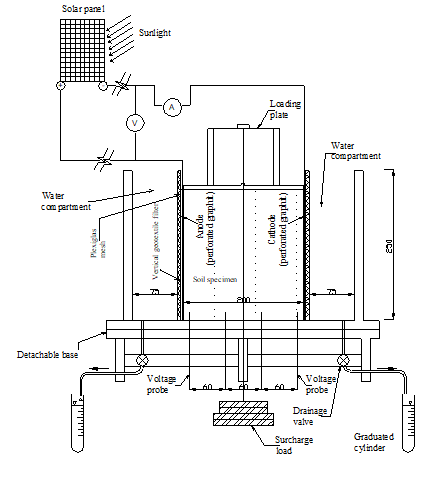
At the end of each test, the soil was extruded from the cell and divided into 5 equal sections, S1, S2, S3, S4, and S5 between the anode and the cathode. The soil from each section was tested for water content, copper concentration, and pH. Part of the soil in each section was squeezed and the soil pore fluid was collected. The pH and electrical conductivity of the pore soil fluid were determined, and the copper concentration was obtained using inductivity couple plasma-optical emission spectrometry (ICP-OES). To determine the copper concentration and pH at each of the five sections, a soil sample was air-dried for 48 to 72 hr and then grounded. The pH in the dry soil was measured with a pH meter by mixing 5 g of dry soil with 10 ml of deionizer water. For analysis of copper concentration, 2.2 g of dry soil was mixed with 11 ml of concentrated nitric acid. The mixture was agitated in a digital shaker for 1 hr at 150 rpm. Afterward, the mixture was centrifuge for 20 min at 5000 rpm. The concentration of copper in the supernatant was then determined using ICP-OES. It must be noted that ratio of clay and sand in the soil samples tested after the remediation process was kept at 2:1 similar to before the remediation.
4. Results and Discussion
4.1. Applied Voltage and Electric Current Equations
Figure 2 shows the profile of applied voltage and electric current during the remediation for the four electrokinetic cells. The zero values are corresponding to the night time. The applied voltage and electric current vary during the daytime according to the degree of lightness/ brightness. As seen in the figure, for all cells, the applied voltage and the current had values rapidly increased in the morning after the sunrise (i.e. 6 am), and peak values were reached during the day between 10 am and 4 pm then decreased towards the end of the day at sunset. The output potential of solar cells was zero in darkness and therefore the voltage and the current were diminished. From Figure 2, the maximum applied voltage (41 V) during the test period of seven days was found to be at the peak hours. At the period of maximum applied voltages, the corresponding maximum current values (0.511, 0.398, 0.385, and 0.383 A, for the homogenous clay, clay-sand layers, clay-sand mixture, and clay with sand pockets, respectively) were found to be on the first day of remediation and the minimum values (0.147, 0.167, 0.058, and 0.106 A, respectively) were reported on the last day of remediation.
The decrease in current resulted from the decrease in electrical conductivity of the soil during the remediation process [12-16]. The change in the conductivity of soil during an electrokinetic process is a result of two opposing mechanisms. The bulk electrical conductivity of a soil is a product of the electrical conductivity of the two components of the soil, namely, the soil pore fluid (water) and soil solids [17-19].
In general, the electrical conductivity of the pore fluid is much higher than that of the soil solids and thereby dominates the bulk conductivity of the soil. Therefore, as water is drained out during an electrokinetic remediation process, the bulk electrical conductivity of the soil decreases. However, for water still remaining inside the soil pores, the electrical conductivity increases with the remediation time as a result of electrolytic reactions associated with the electrokinetic process [13-16]. Therefore, as the drainage of water during an electrokinetic remediation deceases with time, the increase in the electrical conductivity of the pore fluid by the electrolytic reactions may become more dominant than the decrease in soil conductivity resulting from the draining of water. Thus, the bulk conductivity of the soil and thereby the electric current through the soil may increase during the remediation.
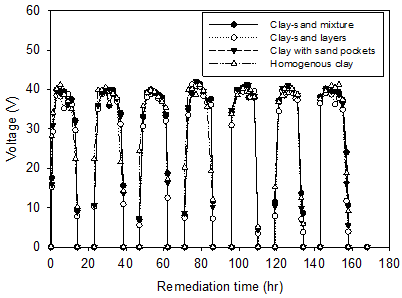
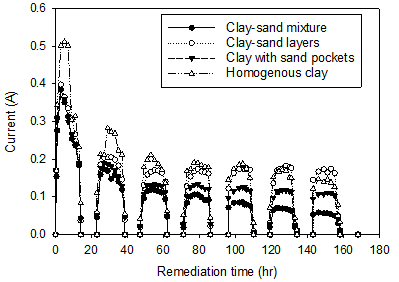
4.2. Water Collected and Water Content
The cumulative volume of water drained from the soil during the electrokinetic remediation is shown in Figure 3. As seen in the figure, cumulative volumes of 1024, 875, 667, and 519 ml were collected by the end of the electrokinetic remediation from the homogenous clay, clay-sand mixture, clay with sand pockets, and clay-sand layers, respectively. The larger volume collected in the homogenous clay may be attributed to the higher electric current observed during the test and therefore the highest power consumptions. The least volume collected from clay-sand layers may be imputed to the low water content of the sand layer. As the sand layer may have lost it water content during the early hours of the test, the drained water was primarily from the clay layers during most of the remediation. Moreover, the electrical conductivity of the sand layer would have significantly decreased during the early hours of remediation as the water content decreased further reducing the water removal efficiency.
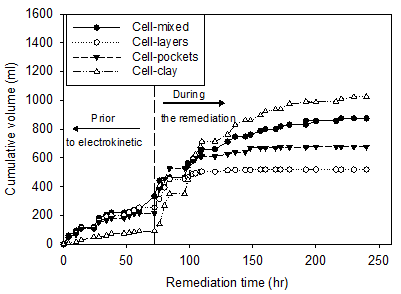
Figure 3 shows the volume of water collected during the early hours of remediation, i.e. immediately following the 72 hr of loading, to be higher than that during the late hours of the remediation. This is to be expected as more water was available at the start of the remediation process. At the end of remediation, the volumes of water collected from the three tests with heterogeneous soils were between 519 to 875 ml. By comparison to the test with homogenous soil (1024 ml), it can be concluded that application of voltage of 41 V was somewhat effective in removing water from heterogeneous soils (51% to 85%). The concentrations of the copper in water collected after electrokinetic remediation in the homogenous clay, clay-sand mixture, clay-sand layers and clay with sand pockets were 28.7 g/l, 25.2 g/l, 44.5 g/l, and 7.6 g/l respectively, representing a negligible amount (< 0.01%) of the initial metal mass. This means while electroosmosis was effective in draining the contaminated soils from water, it was unsuccessful in removing the heavy metal contaminant from the soil.
Figure 4 shows the water content at sections S1, S2, S3, S4, and S5 along the soil specimen. D is the horizontal distance between the mid of the layer and the anode and Do is the total length of the soil samples. Thus, the water content at 0.1 D/Do represents the layer near the anode (S1) while at 0.9 D/Do represents the layer near cathode (S5). Although electroosmosis flow was responsible of dewatering the cells, the movement of hydrogen and hydroxyl ions towards the oppositely charged electrode also may affect the water content layout throughout the cell.
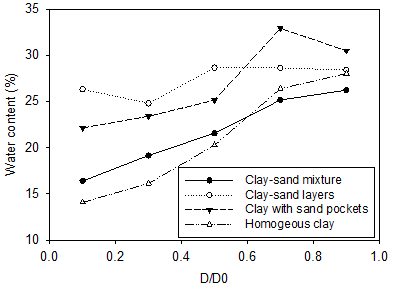
Figure 4 shows an abrupt increase in water content in the sections close to the cathode in most of the tests. For distance up to around 0.6 D/Do, the water content in all the tests was consistent with the volume of water drained from the corresponding cell. On the other hand, the water contents between 0.6 D/Do and 1 D/Do were found to be independent on the volume of water drained. For example, while the least volume of water was drained in the clay-sand layers test, the lowest water content at 0.7 D/Do (60 mm from the cathode) and 0.9 D/Do (20 mm from the cathode) was found in the clay-sand mixture test. As seen in Figure 4, the water content at 0.7 D/Do remained high and roughly similar or slightly higher to that at 0.9 D/Do. The high water content at 0.7 D/Do is a result of the water produced when the acid and base front meet. The acid front generated by electrolysis reactions at the anode travels toward the cathode and the base front produced by the reactions at the cathode travels toward the anode by electromigration (see Figure 5). Due to its smaller size and the direction of movement of water by electroosmosis, typically hydrogen ions travels a longer distance through the soil than hydroxyl ions, resulting in an acid-base meeting closer to the cathode. As they meet, water is formed.
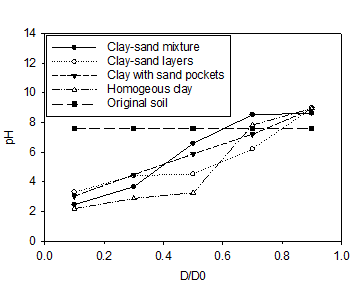
3.3. pH and Heavy Metal Concentration
The initial pH of the clay soil was 7.61. In accordance with electrolysis reactions, the pH profiles for all soils under the tests were acidic near the anode (< 7) and basic in the area close to cathode (> 7). The lowest pH values of 2.2 and 2.47 were reported in the homogenous clay and the clay-sand mixture, respectively. The pH of section S1 in the clay-sand layers and clay with sand pockets specimens were slightly higher than the previous tests. In agreement with electrolysis reactions near the cathode, the highest pH values of were observed at sections S5 and varied between 8.63 and 8.95. Figure 6 shows the concentration of copper along the soil specimen after the electrokinetic remediation. C is the concentration of the copper after the electrokinetic remediation and Co is the initial concentration (150 mg/kg of dry soil). Figures 8 clearly show that the power generated by solar cell panel to drive the electrokinetic remediation was effective in moving copper from the anode toward the cathode. Figure 6(a) shows substantial amounts of copper were removed from sections S1 (87%) and S2 (72%) for clay-sand mixture and precipitated at specimen S3. Figure 6(b), shows that 60% of initial copper was removed from section S1 and about 20% from S2 in clay with sand pockets specimen and most of the removed copper accumulated in section S4. Figure 6(c) shows 57% to 33% of the initial copper was removed from sections S1 to S3 and the removed precipitated in section S5. Figure 6(d) shows appreciable copper was removed from section S1 (86%) and S2 (69%) for the homogenous clay. Little copper was also removed from S3 (16%). All the removed copper precipitated in S4.
By comparing the Figures 5 and 6, it is obvious that copper precipitated near or at the acid-base front junction. The copper removal from the clay with sand pockets and clay-sand layers was not as high as that of the other tests, yet this study shows that electorkinetics can be effective in removing copper from parts of heterogeneous soils. Electroosmosis transport mechanism play the role of dewatering the soil and moving the heavy metal dissolution agent, i.e. H+, toward the cathode resulting in pH profile acidic near the anode and basic near the cathode. In the clay-sand layers and clay with sand pockets tortuosity can cause faster movement of the dissolution agent and consequently less copper removal.
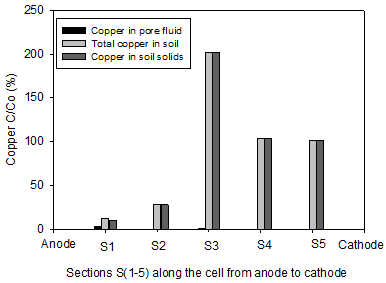
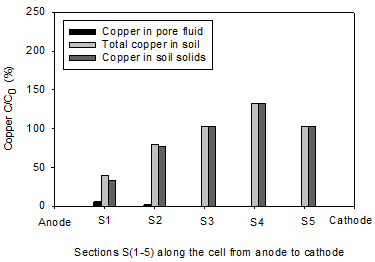
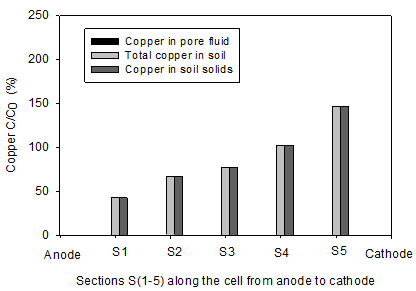
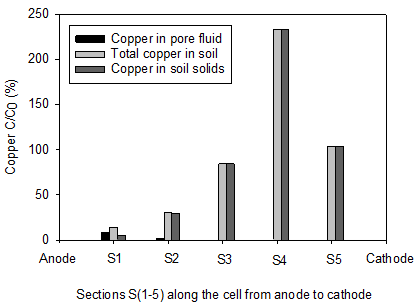
4. LIMITATIONS AND UNCERTAINTIES
The experimental study was carried out in a laboratory scale. Although the results show the effectiveness of electrokinetic in remediating heterogeneous soils, however, the situation investigated did not represent the actual field condition. In addition to that, the effect of the ratio between the clay and sand was not investigated. Also, the effect of the scale in the study’s findings was not identified. In these types of studies scale up may affect the finding. Few to non-similar studies are presented in the available literature. Pilot scale studies are required to investigate the effect of scale in the findings. Changes in the ratio between clay and sand need to be investigated.
5. CONCLUSIONS
An experimental study was carried out to investigate the effectiveness of integrated solar electrokinetic in cleaning heterogeneous soil contaminated with copper to a concentration of 150 mg/kg. The tests were performed in four identical electrokinetic remediation cells. Voltages densities of 205 V/m were applied during 168 hr of testing. The results showed that (1)_ solar cell panels were efficient in generating electric potential for electrokinetic remediation of heterogeneous soils; (2) Electroosmosis flow resulted in removal of 519, 677, 875, and 1024 ml of water from clay-sand layers, clay with sand pockets, clay-sand mixture, and homogenous clay; (3) The pH profile along the cells after electrokinetic remediation was consistent with the electrolysis reactions; (4) Electrokinetic was effective in removing significant amount of copper metal from some of the contaminated soils with the highest removals of 87% in clay-sand mixture test in section S1 near the anode.
Acknowledgements
The research is funded by Natural Science and Engineering Research Council of Canada.
References
[1] Y. B. Acar, R. J. Gale, A. N. Alshawabkeh, R. E. Marks, S. Pupala, M. Bricka, and R. Parker, "Electrokinetic remediation: Basics and technology status," Journal of Hazardous Materials, vol. 40, no. 2, pp. 117-137, 1995 View Article
[2] C. Cameselle, S. Gouveia, and A. Cabo, "Enhanced Electrokinetic Remediation for the Removal of Heavy Metals from Contaminated Soils," in Applied. Science, vol. 11, no. 4, 2021 View Article
[3] B.-G. Ryu, G.-Y. Park, J.-W. Yang, and K. Baek, "Electrolyte conditioning for electrokinetic remediation of As, Cu, and Pb-contaminated soil," in Separation and. Purification. Technology, vol. 79, no. 2, pp. 170-176, Jun. 2011 View Article
[4] M. M. Page and C. L. Page, "Electroremediation of Contaminated Soils," in Journal of Environmental Engineering, vol. 128, pp. 208-219, 2002. View Article
[5] I. Hassan, E. Mohamedelhassan, and E. K. Yanful, "Solar powered electrokinetic remediation of Cu polluted soil using a novel anode configuration," in Electrochimica Acta, vol. 181, pp. 58-67, 2015 View Article
[6] I. Hassan and E. Mohamedelhassan, "Efficacy of Electrokinetics in Remediating Soft Clay Slurries Contaminated with Cadmium and Copper," in Water Air and Soil Pollution, vol. 232, no. 7, p. 289, Jul. 2021, View Article
[7] B.-G. Ryu, G.-Y. Park, J.-W. Yang, and K. Baek, "Electrolyte conditioning for electrokinetic remediation of As, Cu, and Pb-contaminated soil," Separation and Purification. Technology, vol. 79, no. 2, pp. 170-176, Jun. 2011. View Article
[8] M. Pazos, M. Sanromán, and C. Cameselle, "Improvement in electrokinetic remediation of heavy metal spiked kaolin with the polarity exchange technique," in Chemosphere, vol. 62, no. 5, p. 817-822, Feb. 2006. View Article
[9] E. Brauer, "J. Koryta: Ions, Electrodes and Membranes, John Wiley & Sons Ltd., Chichester, New York, Brisbane, Toronto, Singapore 1982. 197 Seiten, Preis: £ 7.90," in Berichte der Bunsengesellschaft für physikalische Chemie, vol. 86, no. 11, pp. 1090-1090, Nov. 1982 View Article
[10] I. Hassan, E. Mohamedelhassan, E. K. Yanful, and Z.-C. Yuan, "Solar power enhancement of electrokinetic bioremediation of phenanthrene by Mycobacterium pallens,"in Bioremediation Journal, vol. 21, no. 2, pp. 53-70, Apr. 2017 View Article
[11] S. Yuan, Z. Zheng, J. Chen, and X. Lu, "Use of solar cell in electrokinetic remediation of cadmium-contaminated soil," Journal of Hazardous Materials, vol. 162, no. 2, pp. 1583-1587, 2009. View Article
[12] I. Hassan, and E. Mohamedelhassan, "Enhancing the Load-Capacity of H-Pile Foundation by Using Electrokinetic Treatment," in International Journal of Civil Engineering. 2023. View Article
[13] I. Hassan, and E. Mohamedelhassan, "Improving the characteristics of a lean clay by electrokinetic treatment," in International Journal of Civil Engineering. 2021 View Article
[14] I, Hassan, E., Mohamedelhassan, E., K., Yanful, and Z-C, Yuan, "Isolation and characterization of novel bacterial strains for integrated solar-bioelectrokinetics of soil contaminated with heavy petroleum hydrocarbons," in Chemosphere, 2019. View Article
[15] I., Hassan, E., Mohamedelhassan, E., K. Yanful, E. K., and M., W., Bo, (2018) "Enhanced electrokinetic bioremediation by pH stabilization," in Environmental Geotechnics. Vol. 5, no. 4, pp. 222-233, 2018, DOI: 10.1680/jenge.16.00001. View Article
[16] I., Hassan, and Mohamedelhassan, E. "Electrokinetic Remediation with Solar Power for a Homogeneous Soft Clay Contaminated with Copper," in International Journal of Environmental Pollution and Remediation, vol. 1, no. 1, pp. 67-74, 2012. View Article
[17] J-C. Xu, Q. Ma, C. Chen, Q-T. Wu, X-X. Long, "Cadmium adsorption behavior of porous and reduced graphene oxide and its potential for promoting cadmium migration during soil electrokinetic remediation," in Chemosphere, vol. 259, 2020. View Article
[18] R. Sprocati, M. Rolle, "Charge interactions, reaction kinetics and dimensionality effects on electrokinetic remediation: A model-based analysis," Journal of Contaminated. Hydrology, vol. 229, 2020. View Article
[19] S. H. Hamdan, G. F. Molelekwa, B. Van der Bruggen, "Electrokinetic Remediation Technique: An Integrated Approach to Finding New Strategies for Restoration of Saline Soil and to Control Seawater Intrusion," ChemElectroChem, vol. 1, no. 7, pp. 1104-1117, 2014. View Article