Volume 8 - Year 2020 - Pages 37-45
DOI: 10.11159/ijepr.2020.005
Decontamination of Automobile Workshop Soils containing Heavy Metals and PAHs using Chelating Agents
Ayodele R. Ipeaiyeda1, Afolarin O. Ogungbemi1,2
1Department of Chemistry, University of Ibadan, Ibadan, Nigeria
ayosade2003@yahoo.com
2Deptartment of Bioanalytical Ecotoxicology, Helmholtz Centre for Environmental Research – UFZ, Leipzig,
Germany
afolarin.ogungbemi@ufz.de
Abstract - Automobile repair workshops are major anthropological sources of polycyclic aromatic hydrocarbons (PAHs) and heavy metals in Nigerian cities. The extent of contamination of soil from workshops in Ibadan city was evaluated by contamination or pollution index (C/P index) assessment. The C/P index assessment indicated that the soils were categorized from moderately contaminated to severely polluted class with Pb, Cd, Cr, Zn and Mn. The concentration of 16 PAHs in the soil samples ranging from 245±21 to 23400±25 µg/kg were far above the levels in the control samples. Washing of soil from different automobile repair workshops using ethtylenediaminetetraacetic acid (EDTA), diethylenetriaminepentaacetic acid (DTPA) and ethanol was investigated. Since mixed contaminants are usually co-existing in the environment, additional experiments involving a combined solution were conducted to remove both PAHs and heavy metals. The results indicated that the removal efficiencies of the extractants were in the order 0.1M DTPA > 0.1M EDTA > 0.01M DTPA > 0.01M EDTA for the heavy metals removal. However, the combined extractants of EDTA and ethanol had much higher PAHs removal efficiency than ethanol alone. The use of mixed extractants was more effective for PAHs and had very little effect for the removal of heavy metals, especially zinc.
Keywords: Chelating agents, Remediation, Soil decontamination, Soil washing
© Copyright 2020 Authors - This is an Open Access article published under the Creative Commons Attribution License terms. Unrestricted use, distribution, and reproduction in any medium are permitted, provided the original work is properly cited.
Date Received: 2020-03-08
Date Accepted: 2020-08-21
Date Published: 2020-12-30
1. Introduction
Environmental pollution is a rampant phenomenon in developing countries where there are no strict regulations to guide anthropogenic activities, resulting in various health risks. Large quantities of pollutants are continuously been introduced into ecosystems as a result of urbanization and industrial processes [1]. Apart from the indiscriminate solid waste disposal on land, other forms of soil polluting activities around automobile repair workshops have been reported [2,3]. The activities occurring in these workshops, where vehicles are maintained and serviced, include the direct soil deposition of solids such as metal scraps and liquid wastes such as fuel and auto-lubricants. Metal scraps containing heavy metals are discarded on bare soil where they could likely leach into underground shallow waters. Most auto-lubricants that spill on soil contain polycyclic aromatic hydrocarbons (PAHs) and have been categorized as probable carcinogens to humans [4]. For this reason, automobile repair workshops have been implicated as a potential source for heavy metals and PAHs’ enrichment of soils arising from auto-lubricant spillage and indiscriminate abandonment of automobile spare parts and tires. During rainfall, these contaminants are washed from soils into the storm-way system and leached into groundwater. Heavy metals and PAHs are of particular interest for study due to their prevalence, toxicity to humans and persistence in the environment [5,6]. Thus soil contaminated with these elements may threaten ecosystem and human health if not decontaminated before reuse of such land.
In Nigeria, various forms of heavy metal pollution have been studied in automobile repair workshops located in cities such as Iwo [7], Port Harcourt [8], Akure [9], Imo river basin [10] and Ibadan [11,12]. Previously in Ibadan, the distribution of the automobile repair workshops across the city was not regulated. As a general practice, automobile workshops were commonly located in residential areas. Recently, however, a new initiative has been put into effect which groups approximately 10 to 20 automobile repair workshops on one site – an arrangement known as an automobile repair workshop village [13]. As can be deducted, the aim of this initiative is to reduce the scattering of these workshops in residential areas. These villages inhabit a variety of auto-mechanics, auto-electricians, vulcanizers and panel beaters. Due to the growing urbanization, many of them are now becoming attractive as high value commercial areas and residential lands. Many land-owners are succeeding in lobbying the state government to give up some of the villages for re-use as residential, recreation and event centers. The reclamation of these automobile repair workshop villages attests to the fact that health and environmental risks are associated with the future use of the villages. It is known that high concentrations of heavy metals and PAHs released into soils may lead to geo-accumulation, and the contaminants can be transported to deep soils, ground-water and other environmental media [14]. This spurred our interest in studying soil contamination at automobile repair workshops in these villages and the extent to which chemical remediation strategy can decontaminate the soils.
Remediation processes are expensive and dependent on the types and levels of contaminants which need to be removed [15,16]. Appropriate remediation of contaminated soil is a major challenge faced by environmental scientists, particularly in cases where soil contains hydrophobic organic compounds. The challenge arises when the organic contaminants are retained in the saturated zone underneath, which make them less bioavailable[17]. For this reason, the natural attenuation of such organic contaminants is very slow, and chemical flushing or washing is often required [18]. One in-situ decontamination technique showing promise over the last decade for organic and inorganic contaminants is phytoremediation because of its promising use of green technology [19,20]. Phytoremediation of heavy metals and PAHs as contaminants is not costly, but time consuming [21]. Further, remediation and bioremediation techniques for removing these contaminants are often very slow or inefficient [22,23]. Hence, an effective remediation technique is required for complex matrices such as soil [24,25]. It is particularly difficult to remediate contaminants possessing low water solubility [26]. New methods are being developed to mobilize these contaminants so as to enhance the performance for removal. Among several processes that have attracted considerable attention for remediation of heavy metals and PAHs contaminated soil is soil washing or flushing with solubilizing agents such as co-solvents [27,28]. Co-solvents are water-miscible organic compounds as a result of their polar structure. Contaminants can be removed by these solvents in two ways, namely by improving the apparent solubility of the contaminant in water, which enhances the mass removal per pore volume, or by decreasing interfacial tension between the water and the contaminant, which may lead to direct mobilization of the contaminant [29]. Several studies on the use of surfactant and chelating compounds for soil remediation have been reported [30–32]. There is dearth of information on the use of mixed extractants such as diethylenetriaminepentaacetic acid (DTPA) and ethylenediaminetetraacetic acid (EDTA) with ethanol for remediation of contaminated soil in Nigeria. In light of this, the objectives of this study were (i) to assess the levels of heavy metals and PAHs in soils from automobile repair workshop villages in Ibadan city (ii) to assess the efficiency of EDTA, DTPA and EDTA with ethanol treatments to remove heavy metals and PAHs respectively from automobile repair workshops soils.
2. Materials and Methods
2.1. Description of Sampling Locations and Sample Collection
Ibadan is the third largest city by population in Nigeria, and is located at 7o 23′ 16′ N latitude and 3o 53′ 47′ E longitude. It has a population of over 3 million people owning personal automobiles and also a high number of public automobiles for commuters that do not own cars. Figure 1 is the map of Ibadan metropolis showing selected automobile repair workshop villages.
Sampling of soil was carried out weekly between November and December, 2014. The samples were collected from nine major automobile repair workshop villages in the city, and the control sample was obtained from the Botanical garden at the University of Ibadan (BGS). Top soil samples (0–15 cm deep) were collected with a hand trowel from each workshop into an aluminium foil for PAHs determination. Another set of samples was collected in the same manner into a set of polythene bag for heavy metal determination. In each case, ten discrete samples were purposefully collected at random from the automobile repair workshop villages and control site. These discrete samples were pooled together to form five composite samples. A total of 250 composite (5 composite sample x 5 weeks x 10 locations) samples were collected for heavy metals and the samples were pooled together for PAHs determination.
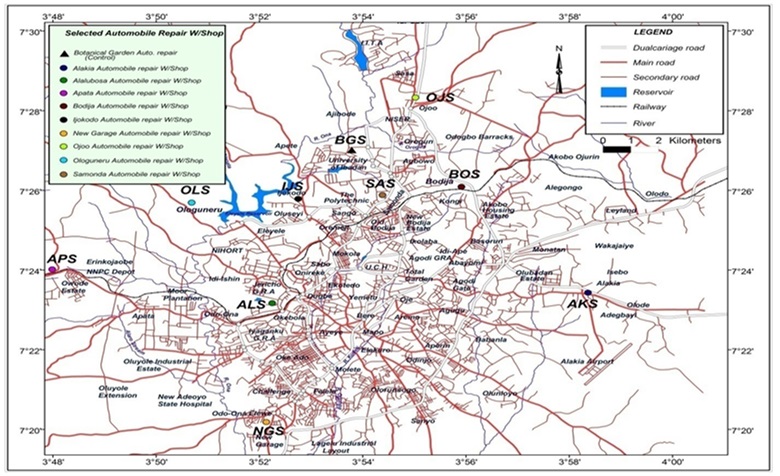
2.2. Soil preparation and analysis
The air dried soil samples were ground and passed through 2 mm mesh sieve. Soil pH was measured in 1:1 soil/water suspension using a calibrated pH meter. Further, organic carbon content was determined by wet oxidation [33]. Particle size analysis was determined using the hydrometer method after digestion by organic matter with hydrogen peroxide [34]. For determining initial environmentally available heavy metals, portions of the samples were digested using a mixture of HNO3 and HCl (1:3), and the initial metal concentrations (Pb, Cr, Cd, Ni, Mn, Zn and Cu) were subsequently determined in the digests by Atomic Absorption Spectrophotometry (AAS).
Other soil samples were extracted for the analysis of initial PAHs concentration using standard analytical protocols described by the Association of Official Analytical Chemists [35]. A 5 g soil sample was extracted with 100 mL of double-distilled hexane and dichloromethane (3:1, v/v) in a sonicator. The extract was dried over anhydrous sodium sulphate and concentrated under a stream of nitrogen gas. The concentrated extract was fractionated into aliphatic and PAH fractions by column chromatography with an alumina column. The aliphatic fraction in the extract was eluted with double-distilled hexane, while the aromatic fraction was eluted with a mixture of hexane and dichloromethane (3:1, v/v). The most polar PAHs were eluted with dichloromethane into a pre-cleaned borosilicate beaker. This fraction was concentrated to 0.1 mL under a stream of nitrogen gas before analysis by GC. The fractions were analyzed for PAHs using a GC (HP 6890, Agilent Technologies, Santa Clara, CA) equipped with a flame ionization detector and ChemStation software (rev. A09.01(1206)). Sample separation was performed using a glass column (VF-5MS; 30 m, 0.25 mm, 0.25 µm). The injector and detector temperatures were 250°C and 350°C respectively, and nitrogen gas was used as the carrier gas at 206.9 KPa. The hydrogen and compressed air pressures were 193.1 KPa and 220.6 KPa respectively. The detection limit was 1.0 µg/g for all PAHs. For recovery study, an appropriate volume of a known concentration of a naphthalene standard (Sigma-Aldrich, St. Louis, MO) was spiked into a contaminated soil sample which had previously been analyzed for PAHs. The procedural and chromatography efficiencies were determined by spiking a surrogate standard (2D chrysene, EPA-M-525-IS, 2.0 mg/mL) into the samples. Recoveries ranging from 83.4% to 94.6% were obtained for the surrogate standard.
2.3. Soil washing with chelating agents for the removal of heavy metals
The removal process was conducted according to the procedure described by Mahvi et al. [36]. Soil samples (1 g dry weight) from automobile workshops and control site were weighed into 50 mL polythene tubes and 10 mL each of 0.1 M EDTA or DTPA were added. The mixtures were agitated at 300 rpm at room temperature for 2 h as the optimum time for metal extraction specified by Mahvi et al. The suspensions were allowed to settle for 1 h and the supernatants were then filtered through 0.45 mm filter paper. The residues were washed with de-ionized water. The supernatants were collected into separate 50 mL flasks and made up to mark with de-ionized water. The concentrations of metals were then measured by Atomic Absorption Spectrophotometry. The effect of concentration on removal efficiency was determined by repeating the procedure with 0.01 M of EDTA and DTPA. This allowed an overall comparison of the efficiency of different concentrations of EDTA and DTPA.
2.4. Soil Washing With Mixed Extractant for Combined Heavy Metals and Pahs Removal
Two sets of removal processes were conducted to assess the effects of different washing solutions. The first involved using a single chelating solution and a combined extractant solution of EDTA and ethanol to remove heavy metals. The second involved using only ethanol and a combined extractant of ethanol and EDTA to remove PAHs. The first soil washing for heavy metals was performed as described above. For PAHs, soil samples (1 g dry weight) were weighed into clean amber glass containers and 10 mL of the single (EDTA) and combined extractant solution (EDTA and ethanol) were separately added. The tubes were agitated using a mechanical shaker at a speed of 300 rpm at room temperature for 24 h. After the shaking process, the tubes were transferred to a centrifuge operated at 7000 rpm for 30 min. The supernatants were filtered through 0.45 mm filter to remove particulate in the solution and the residues were washed by de-ionized water. The supernatants were collected into a pre-cleaned glass vial. Further analysis was carried out using Gas Chromatography Flame Ionization Detector (GC-FID).
3. Results and Discussions
3.1. Physicochemical Characteristics of Soil before Chemical Washing
Soil pH, organic carbon content, soil particle size and initial heavy metal concentrations in soil from automobile workshops in Ibadan city are shown in Table 1. The pH of the background soil was 6.02±0.02 and elevated pH values were obtained for soil from the mechanic workshops. Soil from Alalubosa (ALS) had the highest pH (7.82±0.01), while soil from Apata (APT) had the lowest (6.25±0.01). Contamination of soil with heavy metals can stimulate rising of soil pH level [37]. The pH is a key parameter that controls heavy metal transfer behavior in the soil. At a low soil pH, the competition between H+ and the dissolved metals for ligands becomes increasingly significant [38]. The pH of the soil samples varied from 6.45±0.01 to 7.21±0.01. The slightly acidic to neutral nature of the soils suggests that exchangeable acidity of the soils may be low, possibly since there are less H+ to compete with metal adsorption. Consequently, such neutral soils are expected to retain heavy metals and chemically washing them with extractants as means of remediation technique becomes necessary [39]. Generally, acid rain may influence the pH of the soil and hence alter the mobility heavy metals [40]. However, acid rain has been found not to significantly affect polluted soils due to deposits of metal ions and lower biological activity compared to control sites [41]. Nevertheless, depending on the pH of the acid rain, it is important to consider the influence of acid rain on the mobility of heavy metal pollutants for an adequate remediation strategy.
Soil organic carbon is an important indicator of soil quality that also acts as a storehouse of the plant nutrients [42] and contaminants [43], thereby reducing the amount of chemicals that may enter groundwater or waterways where contamination occurs. In this study, organic carbon contents in soil ranged from 5.99±0.14% to 9.74±0.31%. The range of organic matter was 10.4–16.8%. The effect of the organic species is determined by the solubility of the organic matter. Heavy metals bound to more insoluble organic matter will be less mobile, whereas the formation of soluble metal complexes with soluble organic compounds would enhance their mobility [44].
Soil particle size has also been used in some studies to corroborate the efficiency of remediation in soils. Soils at the various locations investigated are predominantly sandy with contents ranging from 70.5±2.4% to 82.5±4.0%. This predisposes the soils in the study area to mobility of heavy metals. The proportions of clay were in the range of 13.5±1.9 to 21.5±0.5% (Table 1). Sandy soils have a higher inter-particle distance because they lack sticky and plastic properties. High content of sandy soil particles suggests that the chelating washing solution can move freely within the inter-particle pores, thereby enhancing removal of metal from the soil surface [45]. Clay soils, on the other hand, tend to be sticky and easily molded. This observation suggests that soils from the studied areas have a smaller capacity to retain water. Thus the permeability of the soils is improved, leading to greater leaching of contaminants [46].
Table 1: Physicochemical characteristics and initial metal concentrations of top-soils from automobile repair workshops in Ibadan city
Soils from all the automobile repair workshops revealed elevated initial metal concentrations above the background concentrations. In fact, it is evident that anthropogenic activities at the workshops contributed significant levels of heavy metals to the workshop soils. The occurrence of manganese in the soils is traceable to engine oil and diesel oil that contain the metal as an additive [47]. High levels of Pb in soils from the mechanic workshops may be attributed to gasoline and engine oil, which has regularly been spilled in the vicinity of the workshops. The contamination or pollution index (C/P index) proposed by Lacatusu [48] is widely used to assess the contamination or pollution degree of heavy metals in soil. The C/P index is calculated as the ratio of measured concentration of a metal to the country’s regulatory allowable limit for such metal in soil. The Nigeria regulatory allowable limits were 0.8 mg/kg, 85 mg/kg, 140 mg/kg, 437 mg/kg and 100 mg/kg for Cd, Pb, Zn, Mn and Cr respectively [49]. The C/P value less than 1.0 depicts contamination categories, while an index value greater than 1.0 defines various classes of pollution (Table 2). The C/P indexes ranged from 0.01 to 60.4 for Pb, 0.63 to 19.4 for Cd, 0.03 to 1.32 for Cr, 0.07 to 0.87 for Zn and 0.03 to 2.22 for Mn. Based on these indexes, soils from the workshops can be categorized as being moderately contaminated to very severely polluted. The soils from Ijokodo (IJS) and Samonda (SAS) locations were excessively polluted with Pb and severely polluted with Cd respectively. This may be due to the low water solubility and hence low mobility of Pb [12]. Additionally, collection of samples from a fresh deposit of Pb or Cd could result in higher concentration of these metals.
Table 2: Contamination/Pollution (C/P) index values and their significances
3.2. Effect of Chelating Agent Concentrations
The ethylenediaminetetraacetic acid (EDTA) and diethylenetriaminepentaacetic acid (DTPA) can form soluble complexes with free metal ions in the solution [50]. The effect of chelant concentration on the contaminated soil samples was investigated using 0.1 M and 0.01 M each of DTPA and EDTA. Figure 2 reveals that 0.1M DTPA and 0.1M EDTA had higher heavy metal removal efficiency than their corresponding 0.01 M solutions. The removal of the heavy metals from the contaminated soil samples increased with increasing chelant concentration. Ghestem and Bermond [51] also reported a rapid initial release of heavy metals from the contaminated soil into the EDTA extracting solution. The process of chelating agents extracting heavy metals from the soil is probably due to the varying numbers of functional groups or binding sites that are capable of complexing heavy metals [52]. In this study, high removal efficiency (81% for Pb, 38% for Cd, 94% for Zn and 65% for Mn) was achieved using 0.1 M DTPA (Figure 2).
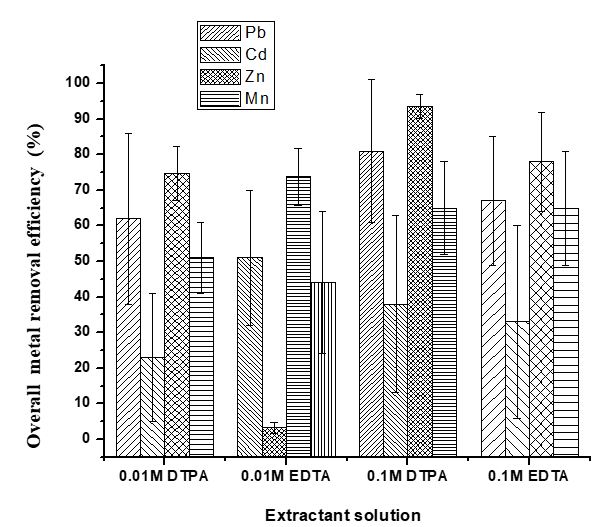
3.3. Comparison of Chelating Agents
The removal efficiency is consistent with the relative stability of the ligand complexes formed by the metal contaminants and the chelating agents [53]. Essentially, when the potential of DTPA as chelating agent was compared with EDTA of the same concentration; it was observed that the DTPA chelant was more effective in removing metal contaminants in the samples than EDTA (Figure 2). DTPA has eight donor sites compared to the six donor sites in EDTA structure. Hence, DTPA forms a higher number of rings which leads to higher stability. This is in consonant with the study on stabilities of various metal-DTPA and metal-EDTA complexes performed by Byegard et al. [54]. However, higher concentration of EDTA had a greater removal efficiency in comparison with DTPA of lower concentrations. The study revealed that the stability generally increases for the individual metal ions following the 0.1 M DTPA > 0.1 M EDTA > 0.01 M DTPA > 0.01 M EDTA order. This trend was observed for Pb, Cd, Zn and Mn in this study.
3.4. Heavy Metals Remediation
It was evident as shown in Figure 2 that Cd had the least removal efficiency compared with other metals. The solubility of Cd appears to be highly dependent on the pH. Cadmium has a low affinity for metal-sorbing phases because its sorption in slightly acidic soil is highly influenced by composition of the liquid phase and competition from Ca2+ and Mg2+ that are possibly present in the soils [55,56]. This finding of low mobility of Cd has Important implication for the development of realistic reclamation techniques for the management of Cd-enriched soils. It is noteworthy that none of the chelators used were capable of mobilizing Cr from the soil samples. It has been considered that Cr III is very stable in soils [57]. Therefore, the immobility of Cr may be responsible for an inadequate Cr removal with the chelators used. However, Choppala [58] observed that a slight mobility of Cr III could occur in soils of lower pH, whereas the adsorption of Cr VI increases. Since the pH of all soils in this study are within the neutral range (6.02-7.50), we can assume that Cr III is the more abundant species in these soils. It was observed that Zn had the highest removal efficiencies in all cases of treatment with DTPA and EDTA solutions (Figure 2). DTPA has removal efficiency for Zn ranging from 74.7 to 93.5% while a range of 73.8% to 78.0% was obtained using EDTA (Figure 2). Many studies of Zn adsorption and retention in soils revealed that Zn can be easily mobilized in soils [59,60]. This suggests that the predominant sand and organic matter of automobile repair soils are not holding Zn strongly, hence the easy solubility of Zn in EDTA and DTPA was evident. Low mobilization of Mn in soil compared with other metal was observed. The low solubility of Mn at pH level near neutrality according to Torres et al. [61] might be responsible for its low mobilization in chelating agent solutions. There is possibility that some metals are bound to organic matrices in the soil. This informed the washing of contaminated soils with mixed extractants (0.1 M EDTA with 100% ethanol, 1:1). Figure 3 shows that mixed extractants have low removal efficiency for Zn in all soil samples compared to a single chelating solution. This low metal removal efficiency observed in mixed extractants may be due to the low dosage (50% lower) of EDTA in the solution.
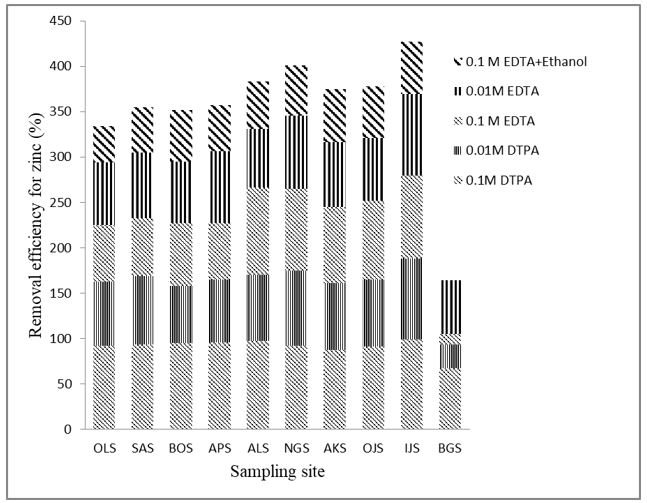
3.5. Polycyclic Aromatic Hydrocarbons Remediation
Polycyclic aromatic hydrocarbons (PAHs) are major contaminants associated with spent engine oil and they are usually deposited on soil surfaces. Table 3 shows the PAHs concentrations before the remediation process for the automobile repair workshop soil (ARWS) and control soil (CTS) samples. 16 PAHs were analyzed and their concentrations in the ARWS ranged from 450 to 234000 µg/kg with naphthalene being the highest while benzo(b)fluoranthene was the lowest. The concentration before remediation for the 16 PAHs in the control sample (CTS) ranged from 0.03 to 12.4 µg/kg with acenaphtelene having the highest concentration while benzo(g,h,i)perylene had the least. It was observed that there was a significant difference between the concentration of PAHs in soils from the automobile repair workshops and the control site. This indicates that soils from automobile repair workshops had been contaminated with PAHs.
Table 3: Concentrations (µg/kg) of PAHs in soils from automobile repair workshops and control site
Two different extractant solutions were used for the removal of PAHs from the soil samples. Ethanol was used as a single extractant, while EDTA (0.1 M) and ethanol were used as a combined extractant. Purposely for this study, EDTA was used as a part of the combined extractant to compare the efficiency of PAHs removal from the soil using a polar organic solvent and the solvent in the presence of a chelant. Figures 4 and 5 show the removal efficiency of the PAHs in automobile repair workshop soils and the control soil sample respectively. It was observed that the removal efficiency of the combined extractant supersedes that of the single extractant for all the 16 PAHs in both automobile repair workshop soils and the control sample. This indicates that ethanol, which is an organic solvent, is less efficient in removing PAHs as compared to a mixture of ethanol and EDTA. This could be attributed to the fact that the solubility of ethanol was increased by the presence of EDTA. Reddy et al. [62], used different extractants including EDTA to remediate PAHs and discovered that EDTA as a single extractant was not able to remove PAHs from the soil.
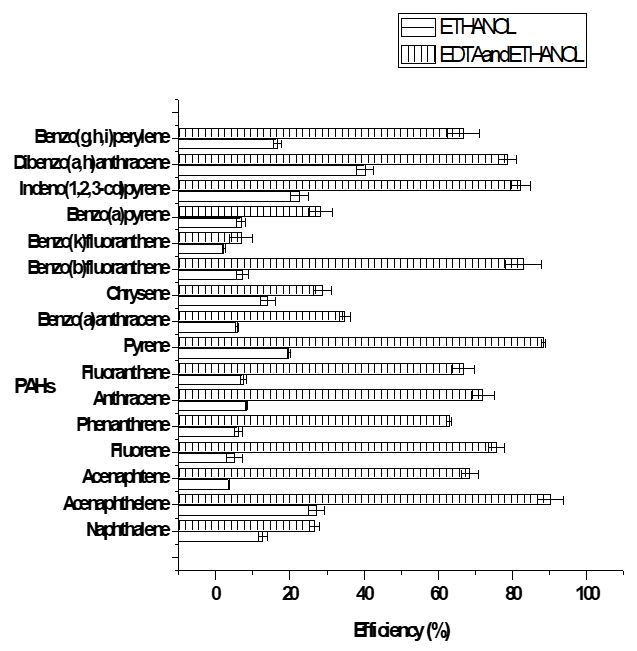
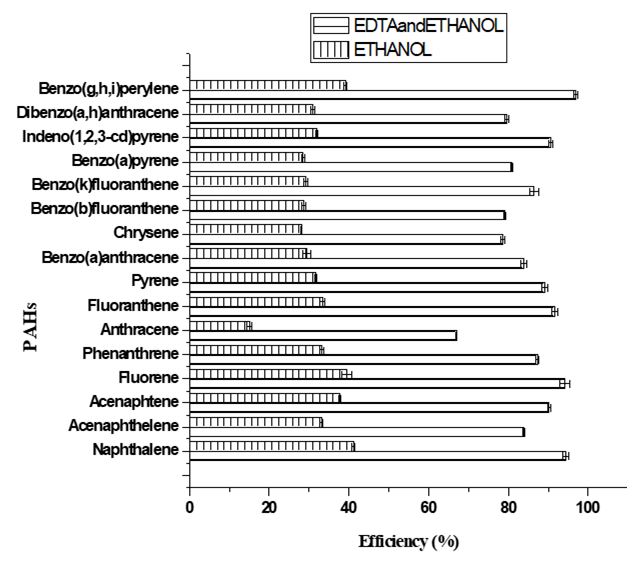
4. Conclusion
In this study, we assessed the levels of heavy metals and PAHs in soils from automobile repair workshops and also the efficiency of EDTA, DTPA and EDTA with ethanol treatments to decontaminate the soils. Concentrations of heavy metals and PAHs found in the soils from all the automobile repair workshops were significantly higher than the background concentrations. This was attributed to the anthropogenic activities at the workshops. Soil washing using chelating agents was employed to remove the contaminants. The best chelant solution was DTPA and the order of remediation efficiency for the metals from the contaminated soil samples was 0.1 M DTPA > 0.1 M EDTA > 0.01 M DTPA > 0.01 M EDTA. The combined extractant (EDTA and Ethanol) had higher remediation efficiency than the single extractant (ethanol) for removing PAHs but was not effective for removing heavy metals alone. This suggests that the use of combined extractant should be encouraged for optimum remediation of organic pollutants such as PAHs in the presence of toxic heavy metals in soil. In conclusion, soil washing may be suitable to decontaminate soils from automobile workshops but the high cost and resources required to excavate the soil before washing could be a disadvantage of this method.
Acknowledgement
We thank Dr. Eberhard Küster for the discussions and comments to improve the paper. We also thank the reviewers for their critical comments.
References
[1] Begum A, Ramaiah M, Khan I, Veena K. Analysis of heavy metals concentration in soil and litchens from various localities of Hosur Road, Bangalore, India. J Chem., vol. 6, no. 1, pp. 13–22, 2009. View Article
[2] Nijenhuis WAS, Van Pul WAJ, De Leeuw F. Deposition of heavy metals to the Convention waters of the Oslo and Paris Commissions. Water Air Soil Pollut., vol. 126, no. 1-2, pp. 121–149, 2001. View Article
[3] Skupinska K, Misiewicz I, Kasprzycka-Guttman T. Polycyclic aromatic hydrocarbons: physicochemical properties, environmental appearance and impact on living organisms. Acta Pol Pharm., vol. 61, no. 3, pp. 233–240, 2004.
[4] Kumar B, Verma VK, Sharma CS, Akolkar AB. Estimation of toxicity equivalency and probabilistic health risk on lifetime daily intake of polycyclic aromatic hydrocarbons from urban residential soils. Hum Ecol Risk Assess An Int J., vol. 21, no. 2, pp. 434–444, 2015. View Article
[5] Brown JN, Peake BM. Sources of heavy metals and polycyclic aromatic hydrocarbons in urban stormwater runoff. Sci Total Environ., vol. 359, no. 1-3, pp. 145–155, 2006. View Article
[6] Xia B, Shen S, Xue F. Phytoextraction of heavy metals from highly contaminated soils using Sauropus androgynus. Soil Sediment Contam An Int J., vol. 22, no. 6, pp. 631–640, 2013. View Article
[7] Ipeaiyeda AR, Dawodu M. Heavy metals contamination of topsoil and dispersion in the vicinities of reclaimed. Bull Chem Soc Ethiop., vol. 22, no. 3, 2008. View Article
[8] Iwegbue CMA. Metal fractionation in soil profiles at automobile mechanic waste dumps. Waste Manag Res., vol. 25, no. 6, pp. 585–593, 2007. View Article
[9] Ilemobayo O, Kolade I. Profile of heavy metals from automobile workshops in Akure, Nigeria. J Environ Sci Technol., vol. 1, no. 1, pp. 19–26, 2008. View Article
[10] Nwachukwu MA, Feng H, Achilike K. Integrated study for automobile wastes management and environmentally friendly mechanic villages in the Imo River Basin, Nigeria. African J Environ Sci Technol., vol. 4, no. 4, 2010.
[11] Onianwa PC, Jaiyeola OM, Egekenze RN. Heavy metals contamination of topsoil in the vicinities of auto-repair workshops, gas stations and motor-parks in a Nigerian city. Toxicol Environ Chem., vol. 84, no. 1–4, pp. 33–39, 2003. View Article
[12] Adelekan BA, Abegunde KD. Heavy metals contamination of soil and groundwater at automobile mechanic villages in Ibadan, Nigeria. Int J Phys Sci., vol. 6, no. 5, pp. 1045–1058, 2011.
[13] Duru CE, Enedoh MC, Duru IA. Physicochemical Assessment of Borehole Water in a Reclaimed Section of Nekede Mechanic Village, Imo State, Nigeria. Chem Africa., vol. 2, no. 4, pp. 689–698, 2019. View Article
[14] Lokeshwari H, Chandrappa GT. Impact of heavy metal contamination of Bellandur Lake on soil and cultivated vegetation. Curr Sci., vol. 91, no. 5, pp. 622–627, 2006.
[15] Lewandowski I, Schmidt U, Londo M, Faaij A. The economic value of the phytoremediation function–assessed by the example of cadmium remediation by willow (Salix ssp). Agric Syst., vol. 89, no. 1, pp. 68–89, 2006. View Article
[16] Khalid S, Shahid M, Niazi NK, Murtaza B, Bibi I, Dumat C. A comparison of technologies for remediation of heavy metal contaminated soils. J Geochemical Explor., vol. 182, pp. 247–268, 2017. View Article
[17] Chu W, Chan KH. The mechanism of the surfactant-aided soil washing system for hydrophobic and partial hydrophobic organics. Sci Total Environ., vol. 307, no. 1–3, pp. 83–92, 2003. doi:10.1016/S0048-9697(02)00461-8 View Article
[18] Song B, Zeng G, Gong J, وآخ. Evaluation methods for assessing effectiveness of in situ remediation of soil and sediment contaminated with organic pollutants and heavy metals. Environ Int., vol. 105, pp. 43–55, 2017. View Article
[19] Roy S, Labelle S, Mehta P, Mihoc A, Fortin N, Masson C, Leblanc R, Châteauneuf G, Sura C, Gallipeau C, Olsen C. Phytoremediation of heavy metal and PAH-contaminated brownfield sites. Plant Soil., vol. 272, no. 1-2, pp. 277–290, 2005. View Article
[20] Vigliotta G, Matrella S, Cicatelli A, Guarino F, Castiglione S. Effects of heavy metals and chelants on phytoremediation capacity and on rhizobacterial communities of maize. J Environ Manage., vol. 179, pp. 93–102, 2016. View Article
[21] Mousset E, Oturan MA, Van Hullebusch ED, Guibaud G, Esposito G. Soil washing/flushing treatments of organic pollutants enhanced by cyclodextrins and integrated treatments: state of the art. Crit Rev Environ Sci Technol., vol. 44, no. 7, pp. 705–795, 2014. View Article
[22] Colombano S, Saada A, Guerin V, Bataillard P, Bellenfant G, Beranger S, Hube D, Blanc C, Zornig C, Girardeau I. Quelles techniques pour quels traitements–Analyse coûts-bénéfices. Rapp Final BRGM-RP-58609-FR. 2010.
[23] Liu L, Li W, Song W, Guo M. Remediation techniques for heavy metal-contaminated soils: principles and applicability. Sci Total Environ., vol. 633, pp. 206–219, 2018. View Article
[24] Kuppusamy S, Thavamani P, Venkateswarlu K, Lee YB, Naidu R, Megharaj M. Remediation approaches for polycyclic aromatic hydrocarbons (PAHs) contaminated soils: Technological constraints, emerging trends and future directions. Chemosphere., vol. 168, pp. 944–968, 2017. View Article
[25] García-Carmona M, Romero-Freire A, Aragón MS, Garzón FJM, Peinado FJM. Evaluation of remediation techniques in soils affected by residual contamination with heavy metals and arsenic. J Environ Manage., vol. 191, pp. 228–236, 2017. View Article
[26] Kim S-S, Kim J-H, Han S-J. Application of the electrokinetic-Fenton process for the remediation of kaolinite contaminated with phenanthrene. J Hazard Mater., vol. 118, no. 1–3, pp. 121–131, 2005. View Article
[27] Pazos M, Rosales E, Alcántara T, Gómez J, Sanromán MA. Decontamination of soils containing PAHs by electroremediation: a review. J Hazard Mater., vol. 177, no. 1–3, pp. 1–11, 2010. View Article
[28] Mao X, Jiang R, Xiao W, Yu J. Use of surfactants for the remediation of contaminated soils: a review. J Hazard Mater., vol. 285, pp. 419–435, 2015. View Article
[29] Mulligan CN, Yong RN, Gibbs BF. Surfactant-enhanced remediation of contaminated soil: a review. Eng Geol., vol. 60, no. 1–4, pp. 371–380, 2001. View Article
[30] Viglianti C, Hanna K, De Brauer C, Germain P. Removal of polycyclic aromatic hydrocarbons from aged-contaminated soil using cyclodextrins: experimental study. Environ Pollut., vol. 140, no. 3, pp. 427–435, 2006. View Article
[31] Ahn CK, Kim YM, Woo SH, Park JM. Soil washing using various nonionic surfactants and their recovery by selective adsorption with activated carbon. J Hazard Mater., vol. 154, no. 1–3, pp. 153–160, 2008. View Article
[32] Rodriguez-Escales P, Sayara T, Vicent T, Folch A. Influence of soil granulometry on pyrene desorption in groundwater using surfactants. Water, Air, Soil Pollut., vol. 223, no. 1, pp. 125–133, 2012. View Article
[33] Nelson DW, Sommers L. Total carbon, organic carbon, and organic matter. Methods of soil analysis: Part 2 chemical and microbiological properties. vol. 9, pp. 539–579, 1983. View Article
[34] Gee GW, Bauder JW. Particle‐size analysis. Methods soil Anal Part 1 Phys Mineral methods., vol. 5, pp. 383–411, 1986. View Article
[35] AOAC (Association of Official Analytical Chemists). Official methods of analysis. Assoc Anal Chem. 1990.
[36] Mahvi AH, Mesdaghinia AR, Naghipoor D. Comparison of heavy metals extractions efficiency in contaminated soils by various concentrations of EDTA. Pakistan J Biol Sci., vol. 8, pp. 1081–1085, 2005. View Article
[37] Kazlauskaitė-Jadzevičė A, Volungevičius J, Gregorauskienė V, Marcinkonis S. The role of pH in heavy metal contamination of urban soil. J Environ Eng Landsc Manag., vol. 22, no. 4, pp. 311–318, 2014. View Article
[38] Gundersen P, Steinnes E. Influence of pH and TOC concentration on Cu, Zn, Cd, and Al speciation in rivers. Water Res., vol. 37, no. 2, pp. 307–318, 2003. View Article
[39] Guo X, Wei Z, Penn CJ, Xu T, Wu Q. Effect of soil washing and liming on bioavailability of heavy metals in acid contaminated soil. Soil Sci Soc Am J., vol. 77, no. 2, pp. 432–441, 2013. View Article
[40] Rechcigl JE, Sparks DL. Effect of acid rain on the soil environment: A review. Commun Soil Sci Plant Anal., vol. 16, no. 7, pp. 653–680, 1985. View Article
[41] Tyler G. Leaching rates of heavy metal ions in forest soil. Water Air Soil Pollut., vol. 9, no. 2, pp. 137–148, 1978. doi:10.1007/BF00280700 View Article
[42] Rattan RK, Datta SP, Chhonkar PK, Suribabu K, Singh AK. Long-term impact of irrigation with sewage effluents on heavy metal content in soils, crops and groundwater—a case study. Agric Ecosyst Environ., vol. 109, no. 3–4, pp. 310–322, 2005. View Article
[43] Watson RT, Noble IR, Bolin B, Ravindranath NH, Verardo DJ, Dokken DJ. Land use, land-use change and forestry: a special report of the Intergovernmental Panel on Climate Change. Cambridge University Press; 2000.
[44] Sekaly AL, Mandal R, Hassan NM, Murimboh J, Chakrabarti CL, Back MH, Gregoire DC, Schroeder WH. Effect of metal/fulvic acid mole ratios on the binding of Ni (II), Pb (II), Cu (II), Cd (II), and Al (III) by two well-characterized fulvic acids in aqueous model solutions. Anal Chim Acta., vol. 402, no. 1–2, pp. 211–221, 1999. View Article
[45] Fijałkowski K, Kacprzak M, Grobelak A, Placek A. The influence of selected soil parameters on the mobility of heavy metals in soils. Inżynieria i Ochr środowiska., vol. 15, pp. 81–92, 2012.
[46] Kujawski W, Koter I, Koter S. Membrane-assisted removal of hydrocarbons from contaminated soils—laboratory test results. Desalination, vol. 241, no. 1–3, pp. 218–226, 2009. View Article
[47] El-Hasan T, Batarseh M, Al-Omari H, Ziadat A, El-Alali A, Al-Naser F, Berdanier BW, Jiries A. The distribution of heavy metals in urban street dusts of Karak City, Jordan. Soil Sediment Contam An Int J., vol. 15, no. 4, pp. 357–365, 2006. View Article
[48] Lacatusu R. Appraising levels of soil contamination and pollution with heavy metals. Eur Soil Bur., vol. 4, pp. 93–102, 2000.
[49] Denneman CAJ, Robberse JG. Ecotoxicological risk assessment as a base for development of soil quality criteria. In Contaminated Soil’90. Springer, pp. 157–164, 1990. View Article
[50] Nowack B. Environmental chemistry of aminopolycarboxylate chelating agents. Environ Sci Technol., vol. 36, no. 19, pp. 4009–4016, 2002. View Article
[51] Ghestem JP, Bermond A. EDTA extractability of trace metals in polluted soils: a chemical-physical study. Environ Technol., vol. 19, no. 4, pp. 409–416, 1998. View Article
[52] Neale CN, Bricka RY, Chao AC. Evaluating acids and chelating agents for removing heavy metals from contaminated soils. Environ Prog., vol. 16, no. 4, pp. 274–280, 1997. View Article
[53] Allen HE, Chen P. Remediation of metal contaminated soil by EDTA incorporating electrochemical recovery of metal and EDTA. Environ Prog., vol. 12, no. 4, pp. 284–293, 1993. View Article
[54] Byegård J, Skarnemark G, Skålberg M. The stability of some metal EDTA, DTPA and DOTA complexes: Application as tracers in groundwater studies. J Radioanal Nucl Chem., vol. 241, no. 2, pp. 281–290, 1999. View Article
[55] Chaney RL, Ryan JA, Brown SL. Environmentally acceptable endpoints for soil metals. Environ Availab soils chlorinated Org Explos Met Am Acad Environ Eng, Annapolis, MD., pp. 111–154, 1999.
[56] Chaney RL, Ryan JA, Umweltschutz F. Risk based standards for arsenic, lead and cadmium in urban soils: Summary of information and methods developed to estimate standards for Cd, Pb and as in urban soils. Dechema Frankfurt, Germany, 1994.
[57] Mukherjee AB. Chromium in the environment of Finland. Sci Total Environ., vol. 217, no. 1–2, pp. 9–19, 1998. View Article
[58] Choppala G, Kunhikrishnan A, Seshadri B, Park JH, Bush R, Bolan N. Comparative sorption of chromium species as influenced by pH, surface charge and organic matter content in contaminated soils. Journal of Geochemical Exploration, vol. 184, pp. 255–260, 2018. doi:10.1016/j.gexplo.2016.07.012 View Article
[59] Voegelin A, Kretzschmar R. Modelling sorption and mobility of cadmium and zinc in soils with scaled exchange coefficients. Eur J Soil Sci., vol. 54, no. 2, pp. 387–400, 2003. View Article
[60] Imran M, Arshad M, Khalid A, Kanwal S, Crowley DE. Perspectives of rhizosphere microflora for improving Zn bioavailability and acquisition by higher plants. Int J Agric Biol., vol. 16, no. 3, 2014.
[61] Torres MA, Gaines RR. Paleoenvironmental and paleoclimatic interpretations of the Late Paleocene Goler Formation, Southern California, USA, based on paleosol geochemistry. J Sediment Res., vol. 83, no. 8, pp. 591–605, 2013.View Article
[62] Reddy KR, Al-Hamdan AZ, Ala P. Enhanced soil flushing for simultaneous removal of PAHs and heavy metals from industrial contaminated soil. J Hazardous, Toxic, Radioactive Waste, vol. 15, no. 3, pp. 166–174, 2010.View Article