Volume 3 - Year 2015 - Pages 27-32
DOI: 10.11159/ijepr.2015.004
Immobilisation of Metals in Contaminated Landfill Material Using Orthophosphate and Silica Amendments: A Pilot Study
Danielle Camenzuli1, Damian B. Gore1, Scott C. Stark2
1Macquarie University, Department of Environmental Sciences,
North Ryde 2109, Sydney, Australia.
Danielle.Camenzuli@mq.edu.au; Damian.Gore@mq.edu.au
2Australian Antarctic Division,
201 Channel Highway, Kingston 7050, Hobart, Australia.
Scott.Stark@aad.gov.au
Abstract - Immobilization and encapsulation of contaminants using silica and orthophosphate based chemical treatments are emerging technologies applicable to the management of metal contaminated soil. While the efficacy of orthophosphate treatment is well documented, there is a paucity of research on the application of silica or coupled orthophosphate and silica chemical treatments to metal contaminated soil. This paper presents a pilot scale bench study on the use of silica and coupled orthophosphate-silica treatments for the immobilization of metal contaminants in soil material obtained from the Thala Valley landfill, East Antarctica, which in places has petroleum hydrocarbons mixed with metal-contaminated sediment. The performance of the treatments trialed was assessed by the concentrations of copper, zinc, arsenic and lead released using the Toxicity Characteristic Leaching Procedure. The results of this pilot study demonstrate that the orthophosphate-silica treatment was the most effective and reduced leachable copper, zinc and lead by 95%, 96% and 99%, respectively, relative to the experimental controls. Further development of this technique will require additional research evaluating its long-term performance under a range of environmental conditions. Studies investigating potential adverse effects of silica and orthophosphate-silica treatments are also necessary, to demonstrate the environmental risk and efficacy of these remediation technologies.
Keywords: Contamination; Remediation; Silica; Orthophosphate; Landfill; Chemical Fixation
© Copyright 2015 Authors - This is an Open Access article published under the Creative Commons Attribution License terms. Unrestricted use, distribution, and reproduction in any medium are permitted, provided the original work is properly cited.
Date Received: 2014-08-29
Date Accepted: 2015-03-13
Date Published: 2015-03-31
1. Introduction
Human activities such as mining, smelting, manufacturing and agriculture have resulted in a broad distribution of metal contaminated sites across the globe (Järup 2003). The legacy and exposure effects of contaminants such as copper, cadmium and lead on the environment and human health are well documented (Järup 2003; Poland et al., 2003; Taylor et al., 2010, 2013), and the management of these contaminated sites remains an ongoing global problem. Efficient management of metal contaminated sites requires the development of cost-effective techniques that are applicable under a range of environmental conditions, and do not engender environmental harm. Technologies being developed to manage metal contaminated land more efficiently include phytoremediation, bioremediation and electrokinetic remediation; however, the efficacy of these techniques relies heavily on favorable environmental conditions (Martin and Ruby 2004). Silica and orthophosphate based immobilization treatments also demonstrate potential for a range of contaminated sites and environments (Mitchell et al., 2000a, b; White et al., 2012).
Orthophosphate immobilization has been trialed successfully in laboratory experiments conducted under cold (2 °C) conditions and through multiple freeze-thaw cycles (Hafsteinsdóttir 2011, 2013; White et al., 2012). However, two concerns remain with orthophosphate treatments. Firstly, over dosage with reagents may lead to orthophosphate contamination of the environment, with the attendant risks of ecosystem disturbance including eutrophication. Secondly, if organic contaminants such as petroleum hydrocarbons have been co-disposed with inorganic waste, organic coatings on contaminants may hinder or even prevent effective reaction with orthophosphate. In this situation, silica amendments offer an alternative approach to contaminant immobilization. Silica treatment can work via direct reaction with contaminants and by microencapsulation, allowing the immobilization of hydrocarbon-coated contaminants. Therefore, its use alone or coupled with orthophosphate, may be far more efficient than treatment with orthophosphate only. A slight excess of silica in the environment is not of great concern in most earth surface environments where silicate rocks and sediments abound, and there is little foreseeable risk of ecosystem disturbance with the dosages to be applied using this method.
Treatment of contaminated soil with orthophosphate or silica requires the application of a powder or solution to contaminated soil (Arocha et al., 1996; Mbhele 2007). Once mixed with soil and water, orthophosphate and silica treatments react with metal cations including Mg2+, Ca2+, Fe3+, Cu2+, Zn2+ and Pb2+ to immobilize metals and precipitate insoluble metal-orthophosphate (White et al. 2012) or metal-silicate (Mitchell et al., 2000a; b; Abdel-Hamid et al., 2012). Contaminants are considered immobilized, and thus less hazardous (Mitchell et al., 2000a, b; Mbhele 2007), once they are transformed into inert or sparingly soluble forms and their leachability is reduced significantly (McDowell 1994; Zhu et al., 2004; Sonmez and Pierzynski, 2005).
While the potential of orthophosphate treatments for remediating metal contaminated soil is well documented (Zhu et al., 2004; Sonmez and Pierzynski 2005; White et al., 2012; Camenzuli et al., 2014), there is a paucity of studies reporting on the efficacy of silica treatments at metal contaminated sites (Camenzuli and Gore 2013). Furthermore, we are not aware of any published studies on the use of coupled orthophosphate-silica treatments for metal immobilization. Therefore, the purpose of this pilot study is to investigate the potential of two silica treatments and one orthophosphate-silica treatment for immobilizing Cu, Zn, As and Pb in contaminated soil. This study will provide a platform for subsequent investigations of these treatments on a wider range of contaminated materials and contaminants, and under varying environmental conditions.
2. Materials and Methods
2.1. Experimental Design
The contaminated soil used in this study was sourced in January 2008 from a stockpile at the Thala Valley waste disposal site at Casey Station, East Antarctica (Stark et al., 2006). Concentrations of leachable Cu, Zn, As and Pb in the soil averaged 1.3 ± 1.0, 2.6 ± 1.8, <0.01 and 3.0 ± 3 .8 mg/L, respectively (Thums et al., 2010).
Three treatments and one untreated control (Table 1) were applied in-duplicate at room temperature to columns loaded with 1 kg of Thala Valley soil sieved to <2 mm using a stainless steel mesh. The columns used in this study adopted a design similar to that described by Vandiviere and Evangelou (1998). The silica treatments were applied by mixing calcium carbonate powder with soil, followed by the application of sodium metasilicate dissolved in Type I (ASTM 2011) reagent water (Milli-Q). The phosphate-silica treatment contained Triple Super Phosphate (TSP), calcined magnesia ('Qmag'), calcium carbonate applied as powder, followed by the application of a sodium metasilicate solution (Table 1). X-ray diffractometry showed Qmag to consist mainly of anhydrite (CaSO4) and quartz (SiO2), with minor calcium montomorillonite (Ca0.2(Al, Mg)2Si4O10(OH).2H2O), bassanite (CaSO4.0.5H2O) and trace amounts of other silicates.
The experiment was performed at room temperature, with each column leached daily with 100 ml of Milli-Q water for 10 days. Leachate samples were collected and stored unacidified (to prevent formation of a silica gel) for later analysis, if required. Six months after application of the silica treatments, two soil samples were obtained from each column. These 16 samples, along with 21 samples of untreated soil collected at the start of the experiment, were extracted according to the Toxicity Characteristic Leaching Procedure (TCLP; US EPA Method 1311; US EPA 1992) and analysed at the Australian Antarctic Division for metals using Inductively Coupled Plasma Optical Emission Spectrometry (ICP-OES). Four composite soil samples from the landfill were also analyzed for total metals by hot nitric acid digestion and ICP-OES at Analytical Services Tasmania.
Table. 1. Composition of silica and silica-phosphate treatments applied to Thala Valley landfill material.
Treatment ID | TSP (g) | Qmag (g) | Sodium metasilicate pentahydrate (g) | Calcium carbonate (g) | Solution volume (ml) |
Control | 0 | 0 | 0 | 0 | 210 |
Silica 1 | 0 | 0 | 70 | 40 | 210 |
Silica 2 | 0 | 0 | 100 | 40 | 300 |
Phosphate-silica | 30 | 20 | 70 | 30 | 210 |
2.2. Analysis of Leachable Metals by Toxicity Characteristic Leaching Procedure (TCLP)
The TCLP simulates contaminant leachability from soil under landfill conditions, and can be used to estimate contaminant mobility or classify contaminated soil for disposal (Scott et al., 2005, 2007). To simplify this procedure, we adopted a scaled-down version of US EPA Method 1311, employing smaller quantities of soil and leachate.
TCLP extractions were performed on the <2.0 mm soil with analytical grade reagents and Type I deionized water. The pH of the soil averaged 8.2 ± 1.2 (n=12). Soil samples (2.5 g) were weighed into 50 ml polypropylene tubes (Sarstedt), mixed with 45 ml 0.10 M sodium acetate (pH 4.9) prepared from glacial acetic acid, 1 M NaOH and deionized water, and extracted for 18 h at 20 ± 1 °C on a rotary sample tumbler. Following filtration using 0.45 µm cellulose acetate syringe filters (Sartorius), the extracts were acidified to pH <2 with concentrated HNO3 and analysed with a Varian 720-ES ICP-OES using standard operating conditions for the analytes. Analytical duplicates returned relative standard deviations for all elements of <0.5%. Analyte recovery was measured using matrix spikes and was >94% for all elements.
3. Results and Discussion
Total concentrations of Cu, Zn, As and Pb in the soil averaged 114 ± 88, 190 ± 100 and 3 ± 1 and 210 ± 170 mg/kg, respectively. The TCLP results (Table 2, Figure 1) demonstrate that the phosphate-silica treatment was the most effective at reducing the leachable concentrations of metals from the landfill material. The orthophosphate-silica treatment reduced Cu, Zn and Pb by 94%, 96% and 99%, respectively, relative to the experimental controls. The silica treatments also reduced Cu and Zn relative to the controls. Silica 2 treatment was the only treatment which did not reduce Pb relative to the controls (Figure 1). Arsenic concentrations were increased by all the treatments (Figure 1), which we attribute to competitive phosphate-arsenate (PO43--AsO43-) and silicate-arsenate interactions in the soil (Peryea 1991; Luxton et al., 2006; Burton and Johnston 2012). The mobilizing effect of phosphate on As represents a major shortcoming of phosphate based remedial techniques (Peryea 1991; Munksgaard and Lottermoser 2013).
Table. 2. TCLP results from experimental controls and treated landfill material (mg/L).
Cu | Zn | As | Pb | |
Control | ||||
Mean | 0.72 | 2.69 | 0.01 | 1.35 |
Range | 0.48-1.02 | 2.24-3.52 | 0.01-0.02 | 0.63-2.68 |
Standard Deviation | 0.25 | 0.57 | 0.00 | 0.95 |
Silica 1 | ||||
Mean | 0.55 | 1.65 | 0.02 | 0.63 |
Range | 0.37-0.71 | 1.52-1.90 | 0.03-0.03 | 0.04-1.57 |
Standard Deviation | 0.13 | 0.18 | 0.00 | 0.51 |
Silica 2 | ||||
Mean | 0.61 | 1.57 | 0.02 | 1.97 |
Range | 0.49-0.83 | 1.50-1.62 | 0.018-0.026 | 1.30-2.34 |
Standard Deviation | 0.15 | 0.05 | 0.00 | 0.56 |
Phosphate-silica | ||||
Mean | 0.04 | 0.12 | 0.03 | 0.02 |
Range | 0.04-0.07 | 0.10-0.21 | 0.031-0.042 | 0.02-0.02 |
Standard Deviation | 0.01 | 0.04 | 0.01 | 0.00 |
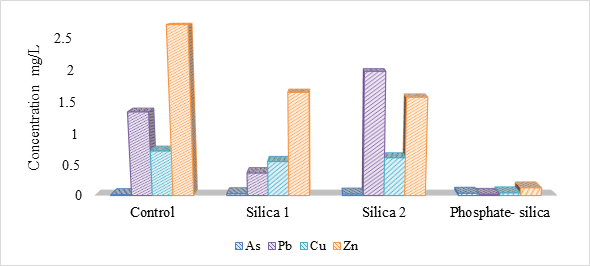
These results demonstrate the potential of silica and phosphate-silica treatments as a technique for remediating metal contaminated land; however, a paucity of research offers only a limited understanding of this approach. The small dataset presented in this paper is based on a small pilot scale experiment with few replicates. This leaves several uncertainties surrounding this technique which warrant further investigation. Of critical importance are long-term studies which evaluate the performance of silica and phosphate-silica treatments under a range of environmental conditions, including the effects of soil pH, oxidation-reduction potential, soil character and temperature on treatment performance, and the stability of minerals and other compounds formed during treatment. Understanding the effect of pH on treatment performance is essential for several reasons. The pH of soil can affect the solubility of treatments (particularly phosphorus based treatments) which can compromise mineral stability (Ma et al., 1993). Secondly, phosphorus release is enhanced in both low and high pH environments and this has important implications for eutrophication (Hafsteinsdóttir et al. 2014). Excessive applications of phosphorus can also acidify the soil and mobilise metals (Hafsteinsdóttir et al. 2014). Although the mobility of Cd, Cu, Sr and Zn in soil decreases with increasing pH, alkaline soil environments can be problematic for soil health, microbial activity and soil structure. Therefore, it is important to balance acidity-alkalinity when applying silica or phosphate-silica mix treatments (Camenzuli and Gore 2013). Furthermore, a study which compares coupled orthophosphate-silica treatments with orthophosphate-only based treatments is essential to distinguish the remedial effects of orthophosphate from silica, and ideally would examine a wider range of analytes than that presented here. Any potential adverse environmental effects associated with these treatments should also be investigated.
4. Conclusion
This pilot study investigated the potential of silica treatments and a coupled orthophosphate-silica treatment as a remediation strategy for metal contaminated soil. Results for contaminant leachability six months after treatment indicate that the coupled orthophosphate-silica treatment was most effective for Cu, Zn and Pb, but mobilized As. However, this study is not comprehensive enough to validate the safety of this technology. Since uncertainty about the safety of this technique under a range of conditions remains, further studies are required before in situ or on-site application to soil contaminated with metals and metalloids, particularly arsenic, is attempted. These should investigate the long-term effectiveness of the treatments under different environmental conditions. Coupled orthophosphate-silica treatments may be a promising technique for metal contaminated sites if future research is able to validate its long-term safety and reliability.
Acknowledgements
The authors wish to thank the Australian Antarctic Division for financial support (AAS4029), and Geoff Stevens, Kathryn Mumford, Tom Statham, Benjamin Freidman, and Chad Sanders for helpful discussions.
References
[1] Abdel-Hamid M. A., Kamel M. M., Moussa E. M. M., Rafaie H. A., "In-situ Immobilization Remediation of Soils Polluted with Lead, Cadmium and Nickel," Global J. of Environmental Res., vol. 6, pp. 1-10, 2012. View Article
[2] Arocha M. A., McCoy B. J., Jackman A. P., "VOC Immobilization in Soil by Adsorption, Absorption and Encapsulation," J. of Hazardous Materials, vol. 51, pp. 131-149, 1996. View Article
[3] ASTM (American Society for Testing and Materials), "Standard Specification for Reagent Water," ASTM D1193 - 06, 2011. View Article
[4] Burton E. D., Johnston S. G., "Impact of Silica on the Reductive Transformation of Schwertmannite and the Mobilization of Arsenic," Geochemica et Cosmochimica Acta, vol. 96, nol. 1, pp. 134-153, 2012. View Article
[5] Camenzuli D., Freidman B. L., Statham T. M, Mumford K. A., Gore. D. B., "On-site and in Situ Remediation Technologies Applicable to Metal Contaminated Sites in Antarctica and the Arctic: A review," Polar Research, vol. 33, 21522, 2014. View Article
[6] Camenzuli D., Gore D. B., "Immobilization and Encapsulation of Contaminants Using Silica Treatments: A review," Remediation, vol. 24, pp. 49-67, 2013. View Article
[7] Hafsteinsdóttir E. G., White D. A., Gore D. B., Stark S. C., "Products and Stability of Phosphate Reactions with Lead Under Freeze-Thaw Cycling in Simple Systems," Environmental Pollution, vol. 159, pp. 3496-3503, 2011. View Article
[8] Hafsteinsdóttir E. G., White D. A., Gore D. B., "Effects of Freeze-Thaw Cycling on Metal-Phosphate Formation and Stability in Single and Multi-Metal Systems," Environmental Pollution, vol. 175, pp. 168-177, 2013. View Article
[9] Hafsteinsdóttir E. G., Fryirs K. A., Stark S. C., Gore D. B., "Remediation of Metal-Contaminated Soil in Polar Environments: Phosphate Fixation at Casey Station, Antarctica," Appl. Geochemistry, vol. 51, pp. 33-43, 2014. View Article
[10] Järup L., "Hazards of Heavy Metal Contamination," Brit. Medical Bulletin, vol. 68, pp. 167-182, 2003. View Article
[11] Luxton T. P., Tadanier C. J., Eick M. J., "Mobilization of Arsenite by Competitive Interaction with Silicic Acid," Soil Sci. Soc. of Amer., 2006. View Article
[12] Ma Q. Y., Traina S. J., Logan T. J., Ryan J. A., "In-situ Lead Immobilization by Apatite," Environmental Sci. and Technol., vol. 27, pp. 1803-1810, 1993. View Article
[13] Martin T. A, Ruby M. V., "Review of In Situ Remediation Technologies for Lead, Zinc, and Cadmium in Soil," Remediation, vol. 14, pp. 35-53, 2004. View Article
[14] Mbhele P. P. "Remediation of Soil and Water Contaminated by Heavy Metals and Hydrocarbons Using Silica Encapsulation," PhD Thesis, University of Witwatersrand, South Africa, 2007. View Article
[15] McDowell T., "Siallon: The Microencapsulation of Hydrocarbons Within a Silica Shell," in Process Engineering for Pollution Control and Waste Minimization, Wise DL, Trantolo DJ ed., Marcel-Dekker Inc., 1994, ch. 19. View Book
[16] Mitchell P., Rybock J. T., Anderson A. L., "Silica Micro Encapsulation: An Innovative Commercial Technology for the Treatment of Metal and Radionuclide Contamination in Water and Soil," in Proc. of the 6th Symposium on Environmental Issues and Waste Management in Energy and Mineral Prod., Calgary, Canada, 2000a.
[17] Mitchell P., Anderson A., Potter C., "Protection of Ecosystem and Human Health via Silica Micro Encapsulation of Heavy Metals," in Proc. of 7th Int. Mine Water Assoc. Congr., Ustron, Poland, 2000b. View Article
[18] Munksgaard N. C., Lottermoser B. G., "Phosphate Amendment of Metalliferous Tailings, Cannington Ag-Pb-Zn Mine, Australia: Implications for the Capping of Tailings Storage Facilities," Environmental Earth Sci., vol. 68, pp. 33-44, 2013. View Article
[19] Peryea F. J., "Phosphate-Induced Release of Arsenic from Soils Contaminated with Lead Arsenate," Soil Sci. Soc. of Amer. J., vol. 55, pp. 1301-1306, 1991. View Article
[20] Poland J. S., Riddle M. J., Zeeb B. A., "Contaminants in the Arctic and the Antarctic: A Comparison of Sources, Impacts, and Remediation Options," Polar Rec., vol. 39, pp. 369-383, 2003. View Article
[21] Scott J., Beydoun D., Amal R., Low G., Cattle J., "Landfill Management, Leachate Generation, and Leach Testing of Solid Wastes in Australia and Overseas," Critical Rev. in Environmental Sci. and Technol., vol. 35, pp. 239-332, 2005. View Article
[22] Snape I., Riddle M. J., Stark J. S., Cole C. M., King C. K., Duquesne S., Gore D. B., "Management and Remediation of Contaminated Sites at Casey Station, Antarctica," Polar Rec., vol. 37, pp. 199-214, 2001. View Article
[23] Stark J. S., Snape I., Riddle M. J., "Abandoned Antarctic Waste Disposal Sites: Monitoring Remediation Outcomes and Limitations at Casey Station," Ecological Manag. and Restoration, vol. 7, no. 1, pp. 21-31, 2006. View Article
[24] Stark S. C., Snape I., Graham N. J., Brennan J. C., Gore D. B., "Assessment of Metal Contamination Using X-ray Fluorescence Spectrometry and the Toxicity Characteristic Leaching Procedure (TCLP) During Remediation of a Waste Disposal Site in Antarctica," J. of Environmental Monitoring, vol. 10, pp. 60-70, 2008. View Article
[25] Sonmez O., Pierzynski G. M., "Phosphorus and Manganese Oxides Effects on Soil Lead Bioaccessibility: PBET and TCLP," Water, Air, and Soil Pollution, vol. 166, pp. 3-16, 2005. View Article
[26] Taylor M. P., Mackay A. K., Hudson-Edwards K. A., Holz E. "Soil Cd, Cu, Pb and Zn Contaminants Around Mount Isa City, Queensland, Australia: Potential Sources and Risks to Human Health," Appl. Geochemistry, vol. 25, pp. 841-855, 2010. View Article
[27] Taylor M. P., Camenzuli D., Kristensen L. J., Forbes M., Zahran S., "Environmental Lead Exposure Risks Associated with Children's Outdoor Playgrounds," Environmental Pollution, vol. 178, pp. 447-554, 2013. View Article