Volume 1 - Year 2012 - Pages 82-89
DOI: 10.11159/ijepr.2012.012
On the Application of High-Resolution Mass Spectrometry to Environmental Analysis
Pedro A. Segura1, Viviane Yargeau1, Christian Gagnon2
1Department of Chemical Engineering, McGill University
3610 University, Montreal, Canada
pedro.segura@mcgill.ca; viviane.yargeau@mcgill.ca
2St. Lawrence Centre, Environment Canada
105 McGill Street, Montreal, Canada
christian.gagnon@ec.gc.ca
Abstract - The occurrence of numerous emerging contaminants such as pharmaceuticals and personal care products in the environment have been reported since they became a "hot topic" in the late 1990's. The detection of these substances in environmental waters is challenging because they are found at very low concentrations and are also structurally heterogeneous. Current analytical techniques used to detect these substances are generally designed to target only a fraction of these compounds and have not yet been adapted to the detection and the identification of unknown substances. It is however crucial to study the presence of transformation products of emerging contaminants that can be potentially found in the environment after biological or chemical degradation. New analytical techniques are thus necessary to screen for the presence of large numbers of suspect emerging contaminants and their degradation products in environmental samples. High resolution-mass spectrometry (HRMS) is a promising technique that has the potential to address the shortcomings of present analytical techniques. Past work with HRMS applied to environmental analysis showed its potential to: i) screen suspect organic contaminants in surface and drinking waters collected in several locations across Canada, ii) screen and confirm the presence of cyanotoxins in algae samples from the Saint-Lawrence River, and iii) elucidate the probable structure of ozonation transformation products of emerging contaminants such as 17α-ethinylestradiol. The advantages and disadvantages of HRMS are discussed here along with strategies proposed in order to further improve its performance.
Keywords: High-Resolution Mass Spectrometry, Emerging Contaminants, Transformation Products, Pesticides, Ozonation, Non-Targeted Substances
© Copyright 2012 Authors - This is an Open Access article published under the Creative Commons Attribution License terms. Unrestricted use, distribution, and reproduction in any medium are permitted, provided the original work is properly cited.
1. Introduction
The presence of contaminants of emerging interest, such as pharmaceuticals and personal care products in environmental waters have been frequently reported in the scientific literature in the last 15 years (Monteiro and Boxall 2010). Our capacity to detect ultra-trace amounts (< 100 ppb) of these substances has been due in part to improvements in analytical instrumentation. For example, in the case of liquid chromatography-mass spectrometry (LC-MS) the limits of detection have been lowered by a factor of 104 in the last 20 years (Barceló and Petrović 2007). However, technology development is not the sole factor. Analytical methodology has also played a major role. In the preface of Advances in the Identification and Analysis of Organic Pollutants in Water (Keith 1981), Lawrence H. Keith wrote a few lines that could be considered nowadays almost prophetic:
"There still remains much to be learned about the kinds of pollutants in water and the chemistry involved. Until we have the correct methodology for analyzing organic compounds, we will continue to miss some even though they maybe literally right under our noses."
One can say that emerging contaminants such as pharmaceuticals have been present in the environment since they were commercially available. In the case of some substances such as ibuprofen, introduced in 1969 (Rainsford et al. 1997), for more than 40 years. However, just as Keith foretold it, the occurrence of ibuprofen in the environment was not reported until 1998 (Ternes 1998) and in Canada until 2003 (Metcalfe et al. 2003). So even today, in spite the powerful analytical instruments available to scientists many more organic contaminants may still go undetected. Why? Because the large majority of analytical methods of detection of these substances are target methods, i.e. they only detect what they are designed to look for. Ultra-trace target methods, based in low-resolution mass spectrometry (LRMS), are designed to preserve only a small amount of information about the composition of the sample; the rest of this analytical information being lost.
Another limitation of the conventional methods of analysis based on LC-MS, is that contrary to gas chromatography-mass spectrometry (GC-MS), databases for the identification of small molecules are still in development. Presently GC-MS libraries allow the identification of more than 212 000 different compounds (National Institute of Standards and Technology 2011), however similar searchable databases for LC-MS contain far fewer entries (<65 000 in MetLin and <15 000 in MassBank as of December 2012). One of the main reasons for the late development of such LC-MS databases is the lack of reproducibility of collision-induced dissociation (CID) spectra which is sensitive to instrument design and operation parameters such as mobile phase composition, flow rate, organic modifiers, etc. (Hough et al. 2000; Josephs and Sanders 2004).
For this reason, high-resolution mass spectrometry (HRMS) is a promising technique that has the capacity to fill the gaps of present ultra-trace target methods based on LRMS. In HRMS, ions are measured at a high resolving power i.e., ions with slightly different m/z ratios can be distinguished from one another (Price 1991; Watson and Sparkman 2007). While there is no precise value of the resolving power necessary to consider a mass measurement as "high resolution", it is generally accepted that acquisition of mass spectra with a resolving power measured at full with half-maximum (RFWHM) > 5 000 should be considered as HRMS (Gross 2004). Mass spectrometers such as the time-of-flight (TOF), the Fourier transform-ion cyclotron resonance (FT-ICR) and the Orbitrap are HRMS instruments since they are capable of reaching RFWHM > 20 000 in the range of m/z300-400 (Krauss et al. 2010). The most important feature of HRMS is the capacity to determine molecular formulas from accurate mass measurements in complexes matrices. HRMS is not absolutely necessary to perform such measurements (Bristow 2005), however this technique becomes highly advantageous when working with complex matrices which may contain many isobaric interferences (same nominal mass but different exact mass) as it is the case in environmental analysis.
Other authors have discussed the trends and emerging application of HRMS to the analysis of micropollutants (Hogenboom et al. 2009; Krauss et al. 2010; Petrović et al. 2010). There are examples in literature of the application of this technique to the field of environmental analysis such as screening (Nurmi et al. 2012) or determination (Farré et al. 2008) of emerging contaminants in wastewaters or surface waters and detection and identification of transformation products (Vanderford et al. 2008). The objective of this manuscript is to clearly demonstrate the potential of HRMS for environmental analysis using several examples of application of the authors' research. Advantages and disadvantages of this technique are also examined and approaches and strategies are proposed in order to improve current analytical performance and streamline data analysis.
2. Methods
2.1 Sample Preparation
Sample preparation is described elsewhere (Lajeunesse et al. 2012; Larcher et al. 2012; Segura et al. 2011). Briefly for the rapid screening of suspect organic contaminants, drinking and surface waters samples were collected from rural and urban locations across Canada distant from point sources of contamination such as wastewater treatment plants. Samples (500 mL) were extracted in triplicate with Oasis HLB solid-phase extraction cartridges (500 mg), evaporated to near dryness and reconstituted to 500 µL (Segura et al. 2011). For the screening and confirmation of cyanotoxins, freeze-dried samples of the alga Lyngbya wollei (20-50 mg, dry mass) were ground and extracted by ultrasonication with 1 mL of 0.1 M acetic acid in 50% methanol-50% water. Extracted samples were then evaporated to dryness and reconstituted to 500 µL (Lajeunesse et al. 2012). Samples used for the structural elucidation of ozonation transformation products were prepared by adding 500 µg L-1 of O3(aq) to a solution containing 250 µg L-1 of 17α-ethinylestradiol and 250 µg L-1 of 17α-ethinylestradiol, 2, 4, 16, 16-d4. Samples were then preconcentrated 200 times by solid-phase extraction prior to analysis (Larcher et al. 2012).
2.2. Sample Analysis
All experiments were carried out using liquid chromatography-high resolution mass spectrometry performed with an Agilent 6530 Accurate-Mass QTOF mass spectrometer coupled to a 1200 series LC system of the same manufacturer. For the screening of suspect organic contaminants and the analysis of cyanotoxins, electrospray ionization (ESI) in the positive mode was used. For the structural elucidation of ozonation transformation products, atmospheric pressure chemical ionization (APCI) in the negative mode was employed. Details about the specific parameters of each application are found elsewhere (Lajeunesse et al. 2012; Larcher et al. 2012; Segura et al. 2011). The "Find by Molecular Feature" function of the MassHunter Qualitative Analysis software was used to perform data processing. This function uses an algorithm based on chromatography parameters (relative height, retention time) as well as on mass spectrometry parameters (exact mass, isotopic abundance and isotope peak spacing) to assign a score (probability match) to the unknown compounds studied. Validation experiments (Segura et al. 2011) showed that a score ≥ 80 was the minimum threshold to avoid false negatives while limiting the number of false positives. Once the signal is identified, the presence of the compound was confirmed by MS/MS experiments or by comparison with the retention time of a standard.
3. Results
3.1 Rapid Screening of Suspect Organic Contaminants
HRMS was applied to screen the presence of organic contaminants that could be potentially found in drinking and surface waters. In this type of non-target method, the mass spectrometer is set to acquire high-resolution mass spectra (resolving power > 10 000) in a large mass range (e.g.m/z 100-1500 ) that results in a multitude of signals being recorded in the acquisition files simultaneously. Not all the signals recorded in these files are relevant, as it is the case of background signals or of contaminants due to sample manipulation. Therefore in order to perform a suspect screening (also called post-target screening), a database of compounds is used to perform a search in the acquisition files according to predefined parameters such as mass accuracy, isotopic pattern, signal intensity, etc. (Segura et al. 2011). This approach is much less work-intensive.
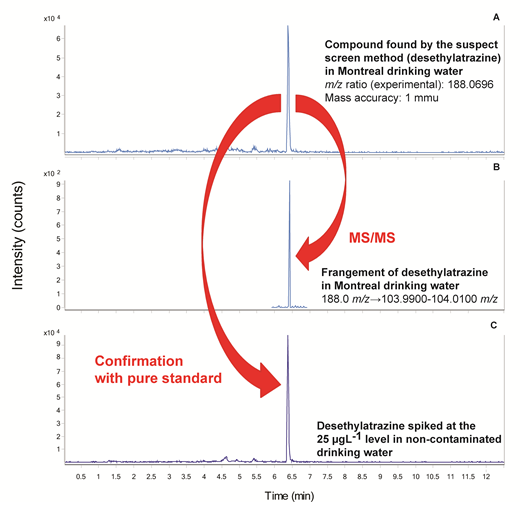
and more effective than examining the most intense signals in an attempt to identify unknowns since many compounds may share the same molecular formula but have different molecular structures, i.e. they are structural isomers (Segura et al. 2011). In addition, not all the substances found in the samples might be environmentally relevant. Therefore, while this approach still targets specific compounds, it relies on a database which includes contaminants of interest selected for their known or suspected toxicity or their potential to be present in the environment at high concentration, e.g. high production volume chemicals. Also, one of the many advantages of suspect screening methods is that the suspect database can be updated and data reprocessed in the future when the awareness of a novel contaminant becomes "emergent". Application of a suspect screening method to drinking and surface water samples collected in urban and rural settings across Canada, revealed that from the 246 common water organic contaminants present in the database (containing pesticides, pharmaceuticals and personal care products among others) at least one organic contaminant was found in 5 out of 11 drinking water samples and in 3 out of 5 surface water samples. The drinking water sample with the highest number of matches (five compounds) was the Montreal tap water (Figure 1). Suspect contaminants found in this sample with a high score (≥ 80) were: desethylatrazine (a degradation product of simazine and atrazine), simazine (herbicide), atrazine (herbicide), 2-aminobenzimidazole (fungicide)
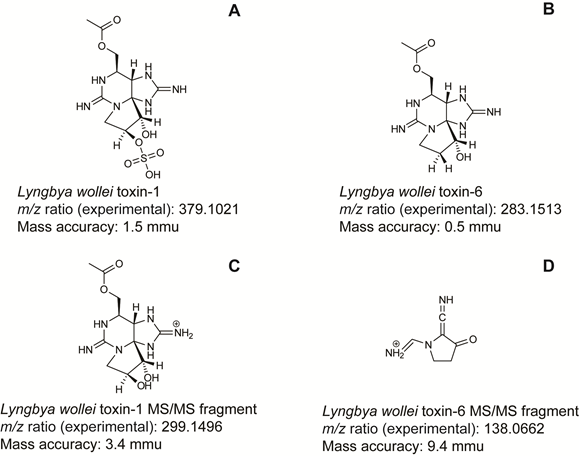
and trenbolone (steroid). Of the surface water samples analyzed, the one collected from the Saint-Lawrence River had the highest number of matches with a score ≥ 80: 2-aminobenzimidazole (fungicide), desethylatrazine, simazine, atrazine and butyl benzyl phtalate (plasticizer). However, only the presence of desethylatrazine, simazine and atrazine could be confirmed in these samples by comparison to standards. These three compounds have been previously quantified in surface and drinking waters by target methods in the region of Montreal (Garcia-Ac et al. 2009).
3.2 Screening and Confirmations of Cyanotoxins
A similar approach was also applied to the confirmation and screening of cyanotoxins produced by the filamentous benthic cyanobacteria Lyngbya wollei (Lajeunesse et al. 2012). In order to screen the presence of cyanotoxins in the samples an in-house database containing 46 hepatotoxins and neurotoxins was used with the MassHunter Qualitative Analysis software. Results showed that the samples did not contain any microcystins or cylindrospermopsins and only 5 cyanotoxins had a high score (> 80): dihydroanatoxin-a, hydroxyhomoanatoxin-a(S/R), L. wollei toxin-1, L. wollei toxin-5 and L. wollei toxin-6. Tandem mass spectrometry (MS/MS) experiments confirmed the presence L. wollei toxin- 1 and L. wollei toxin-6 in the algae extracts.
In the experiments performed by Lajeunesse et al. (2012), a target method had already quantified L. wollei toxin-1 in algae samples, however using the accurate mass measurements obtained by HRMS, the presence of this cyanotoxin was confirmed with a higher degree of certainty. The detection of L. wollei toxin-6 is also a very good example of the potential of HRMS in environmental analysis. Since no analytical standard is available for this cyanotoxin, no target method based on conventional approaches could be developed to detect it. However, using a non-target approach this saxitoxin analogue (L. wollei toxin-6) was found and confirmed with a high degree of confidence (Figure 2).
3.3 Structural Elucidation of Ozonation Transformation Products
HRMS was also applied to elucidate the structure of ozonation transformation products of the synthetic estrogen, 17α-ethinylestradiol. Contrary to biological modifications such as glucoronidation and methylation that can be easily monitored by mass spectrometry, reaction products of ozone with an organic molecule cannot be easily predicted since it involves complex reaction pathways (Ikehata et al. 2006; Masten and Davies 1994) resulting in many cases in the formation of new functional groups such as carboxylic acid, ketone and alhehyde after the cleavage of double bonds or aromatic rings. Experiments showed that after ozonation
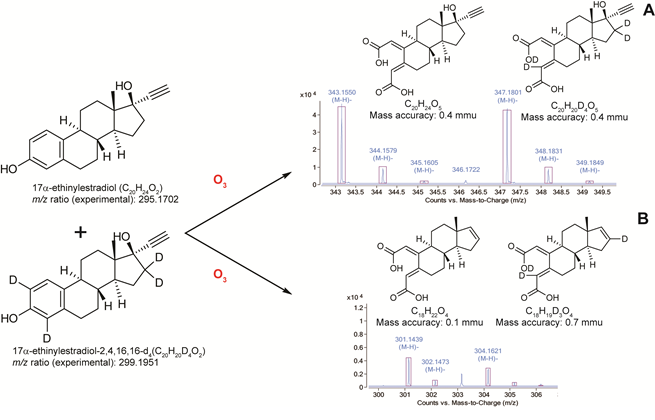
of a pure standard a complex mixture of transformation products was generated. Two ozonation transformation products could be identified with excellent mass accuracy (< 2.03 ppm): one with a mass of 302.1517 u and the other having a higher mass of 344.1628 u (Figure 3A). The corresponding molecular structures were assigned according to structures proposed in previous studies (Vieira et al. 2010). These structures were further confirmed using deuterated standards (Figure 3B), which suggested that the assigned molecular structures were most likely correct.
4. Discussion
The results described previously demonstrate that suspect screening methods using HRMS are a promising tool in the field of environmental science. Coupled with sample enrichment techniques such as solid-phase extraction, they are sensitive enough to detect organic contaminants at the parts-per-trillion level and are not limited by availability of standards or to a few numbers of analytes. By using larger databases, thousands of compounds can be searched in the acquisition files at once, with minimal operator input. However, these methods are still in their infancy and much analytical development is still necessary in order to benefit from the full potential of HRMS. The sample preparation is a key aspect that needs to be improved, since only extracted compounds can be analysed. Therefore solid-phase extraction methods capable of extracting a broader number of substances with high recovery (> 80%) are desirable. The use of other ionization sources such as atmospheric pressure chemical ionization or atmospheric pressure photoionisation might also be necessary since it is unrealistic to expect that all organic contaminants present in a given sample will ionize with the same efficiency under electrospray ionization.
Another key aspect of screening methods that must be improved is the confirmation of potential matches. Since these methods have the potential to identify thousands of compounds in a given sample, it is not practical to confirm them all using pure standards. Also, as it is the case for the cyanotoxins, some analytical standards might not be available. Since MS/MS libraries, which would help identifying compounds using their fragmentation products, are still in development, an interesting avenue is the use of software capable of predicting fragmentation mechanisms. Such software is already available (e.g. HighChem's Mass Frontier and ACD/Labs' ACD/MS Fragmenter) and will certainly emerge as a tool to help better understand the HRMS fragmentation spectra of potential matches, thus reducing the number of false positives.
Concerning the structural elucidation of new contaminants, the advantage of using HRMS for such experiments is that high mass accuracy (< 5 ppm) can be attainable in complex matrices, which has the advantage of reducing the number of possible molecular formulas for a given m/z ratio,
One of the difficulties currently found while attempting the structural elucidation of transformation products is the large number of compounds generated by the various transformation processes (ozonation, photolysis, bacterial metabolism, etc.). Structural elucidation by HRMS could be improved by the integration of ultra-performance liquid chromatography in the method, providing better chromatographic resolution. Better separation could help to reduce spectral interferences and simplify the elucidation process. Also the use of multiple stage mass spectrometry experiments allowing successive MS/MS experiments in specific ions is desirable considering that this approach would further simplify data interpretation. However, in some cases a complete structural elucidation based solely in HRMS is not yet possible with the current technology. The correct spatial distribution of atoms in the molecule requires other powerful techniques such as nuclear magnetic resonance.
5. Perspectives
HRMS instruments do not yet have the sensitivity or dynamic range offered LRMS systems such as the triple quadrupole mass spectrometer (QqQMS) and required for ultra-trace analysis. For these reasons, the QqQMS is presently the best choice for quantification, especially at ultra-trace levels. However, the many advantages of HRMS over LRMS techniques for qualitative analysis suggests that hybrid instruments capable of acquiring spectra at high resolving power such as the quadrupole-TOF spectrometer or the linear ion trap-Orbitrap will be more frequently used in the field of environmental analysis in the near future.
The capacity to perform identification of unknowns based on accurate mass measurements is one of the most powerful applications of this technique, since as mentioned earlier, large searchable LC-MS databases for small molecules are still in development. Unknown identification is further enhanced with MS/MS or multi-stage tandem mass spectrometry (MSn) since fragmentation of precursor ions reveals additional structural information that helps eliminate potential matches. Another of the key applications of HRMS is its capacity to preserve a higher amount of information from the samples than LRMS instruments. In HRMS methods, the detection is performed over a wide m/z range (e.g. 50-1000 m/z) and not at pre-defined m/z values as in LRMS methods. Therefore, the possibility to re-analyse acquisition files to screen for the presence of "new" emerging contaminants is an attractive feature that is not possible when performing target analysis in LRMS instruments.
It is important to stress that now that technological advance has put in the hands of scientists such powerful instruments, emphasis should be given to the correct methodology. Key steps before HRMS analysis such as sample preconcentration and purification, ionization and separation are thus essential to this technique. Sample preconcentration can be improved by using SPE phases of different chemistries such as reversed-phase and ion exchangers in order to maximize analyte retention. Regarding ion formation, other atmospheric pressure ionization techniques should be tested besides ESI such as APCI and atmopsheric pressure photoionization (APPI). The use of multiple ion sources in positive and negative polarity ensures that a larger amount of compounds is being detected. This aspect is particularly important when studying transformation products since the parent compound can ionize very well with one ion source in a given polarity but its transformation products could not. Finally, liquid chromatography remains one of the most important aspects of method development since proper separation facilitates identification and can reduce matrix interferences. Recent techniques such as hydrophilic interaction chromatography (HILIC) offer an interesting alternative over established techniques such as reversed phase liquid chromatography (RP-LC) for the separation of polar compounds.
Just as Lawrence Keith said it more than 30 years ago, the presence of new pollutants will be still missed if the methods used are not appropriate, even with the most advanced analytical instruments. The same applies to the success of HRMS in the field of environmental analysis.
6. Conclusion
The examples given in this manuscript on the application of HRMS to environmental analysis show the potential of this technique to help solve some of the problems faced by many scientists in the field due to the complexity of environmental samples. They showed that HRMS, coupled to proper sample preparation techniques, databases and data processing algorithms, could potentially allow the screening of thousands of compounds simultaneously in a given sample. Also new substances generated during wastewater treatments or in the environment could be identified and this, with a high degree of confidence. While HRMS methods are still in development and instrumentation is still out of reach for most environmental science laboratories, without any doubt HRMS has the potential to become one of the most important analytical techniques in this field of research.
Acknowledgements
This study was supported by the Natural Sciences and Engineering Research Council of Canada (NSERC). We thank Pascal Lemoine, André Lajeunesse and Simone Larcher for their contribution to the various studies presented in this paper.
References
Barceló, D., Petrović, M. (2007). Challenges and Achievements of Lc-Ms in Environmental Analysis: 25 Years On. TrAC, Trends in Analytical Chemistry 26, 2-11. View Article
Bristow, A. W. T. (2005). Accurate Mass Measurement for the Determination of Elemental Formula—a Tutorial. Mass Spectrometry Reviews 25, 99-111. View Article
Farré, M., Gros, M., Hernández, B., Petrović, M., Hancock, P., Barceló, D. (2008). Analysis of Biologically Active Compounds in Water by Ultra‐Performance Liquid Chromatography Quadrupole Time-of-Flight Mass Spectrometry. Rapid Communications in Mass Spectrometry 22, 41-51.View Article
Garcia-Ac, A., Segura, P . A., Viglino, L., Fürtös, A., Gagnon, C., Prévost, M., Sauvé, S. (2009). On-Line Solid-Phase Extraction of Large-Volume Injections Coupled to Liquid Chromatography-Tandem Mass Spectrometry for the Quantitation and Confirmation of 14 Selected Trace Organic Contaminants in Drinking and Surface Water. Journal of Chromatography, A 1216, 8518-8527. View Article
Gross, J. H. (2004). Isotopes. In: Mass Spectrometry: A Textbook. Berlin, Germany:Springer, 67-110..
Hogenboom, A., van Leerdam, J., de Voogt, P. (2009). Accurate Mass Screening and Identification of Emerging Contaminants in Environmental Samples by Liquid Chromatography-Hybrid Linear Ion Trap Orbitrap Mass Spectrometry. Journal of Chromatography, A 1216, 510-519. View Article
Hough, J. M., Haney, C. A., Voyksner, R. D., Bereman, R. D. (2000). Evaluation of Electrospray Transport Cid for the Generation of Searchable Libraries. Analaytical Chemistry 72, 2265-2270. View Article
Ikehata, K., Naghashkar, N. J., El-Din, M. G. (2006). Degradation of Aqueous Pharmaceuticals by Ozonation and Advanced Oxidation Processes: A Review. Ozone: Science and Engineering 28, 353-414. View Article
Josephs, J. L., Sanders, M. (2004). Creation and Comparison of Ms/Ms Spectral Libraries Using Quadrupole Ion Trap and Triple‐Quadrupole Mass Spectrometers. Rapid Communications in Mass Spectrometry 18, 743-759. View Article
Keith L.H. (1981). Preface In: Advances in the Identification and Analysis of Organic Pollutants in Water, Vol. 1, (Keith LH, ed). Ann Arbor, MI:Ann Arbor Science Publishers, Inc., 1190.
Krauss, M., Singer, H., Hollender, J. (2010). Lc–High Resolution Ms in Environmental Analysis: From Target Screening to the Identification of Unknowns. Analytical and Bioanalytical Chemistry, 397, 943-951. View Article
Lajeunesse, A., Segura, P. A., Gélinas, M., Hudon, C., Thomas, K., Quilliam, M. A., Gagnon, C. (2012). Detection and Confirmation of Saxitoxin Analogues in Freshwater Benthic Lyngbya Wollei Algae Collected in the St. Lawrence River (Canada) by Liquid Chromatography-Tandem Mass Spectrometry. Journal of Chromatography, A 1219, 93-103. View Article
Larcher, S., Delbès, G., Robaire, B., Yargeau, V. (2012). Degradation of 17α-Ethinylestradiol by Ozonation—Identification of the by-Products and Assessment of Their Estrogenicity and Toxicity. Environmental International 39, 66-72. View Article
Masten, S. J., Davies, S. H. R. (1994). The Use of Ozonation to Degrade Organic Contaminants in Wastewaters. Environmental Science and Technology, 28, 180-185. View Article
Metcalfe, C. D., Koenig, B. G., Bennie, D. T., Servos, M., Ternes, T. A., Hirsch, R. (2003). Occurrence of Neutral and Acidic Drugs in the Effluents of Canadian Sewage Treatment Plants. Environmental Toxicology and Chemistry 22, 2872-2880. View Article
Monteiro, S., Boxall, A. (2010). Occurrence and Fate of Human Pharmaceuticals in the Environment. Reviews of Environmental Contamination and Toxicology 202, 53-154. View Article
National Institute of Standards and Technology. 2011. Nist Standard Reference Database 1a - User's Guide. View Article
Nurmi, J., Pellinen, J., Rantalainen, A. L. (2012). Critical Evaluation of Screening Techniques for Emerging Environmental Contaminants Based on Accurate Mass Measurements with Time‐of‐Flight Mass Spectrometry. Journal of Mass Spectrometry, 47, 303-312. View Article
Petrović, M., Farré, M., De Alda, M. L., Perez, S., Postigo, C., Köck, M., Radjenovic, J., Gros, M. Barcelo, D. (2010). Recent Trends in the Liquid Chromatography–Mass Spectrometry Analysis of Organic Contaminants in Environmental Samples. Journal of Chromatography A 1217, 4004-4017. View Article
Price, P. (1991). Standard Definitions of Terms Relating to Mass Spectrometry. Journal of the American Society for Mass Spectrometry, 2, 336-348. View Article
Rainsford, K., Roberts, S., Brown, S. (1997). Ibuprofen and Paracetamol: Relative Safety in Non-Prescription Dosages. Journal of Pharmacy and Pharmacology, 49, 345-376. View Article
Segura, P. A., MacLeod, S. L., Lemoine, P., Sauvé, S., Gagnon, C. (2011). Quantification of Carbamazepine and Atrazine and Screening of Suspect Organic Contaminants in Surface and Drinking Waters. Chemosphere 84, 1085-1094. View Article
Sleno, L., Volmer, D. A., Kovaevi, B., Maksi, Z. B. (2004). Gas-Phase Dissociation Reactions of Protonated Saxitoxin and Neosaxitoxin. Journal of the American Society for Mass Spectrometry 15, 462-477. View Article
Ternes, T. A. (1998). Occurrence of Drugs in German Sewage Treatment Plants and Rivers. Water Research 32, 3245-3260. View Article
Vanderford, B. J., Mawhinney, D. B., Rosario-Ortiz, F. L., Snyder, S. A. (2008). Real-Time Detection and Identification of Aqueous Chlorine Transformation Products Using Qtof Ms. Analytical Chemistry 80, 4193-4199. View Article
Vieira, K., Nascentes, C., Augusti, R. (2010). Ozonation of Ethinylestradiol in Aqueous-Methanolic Solution: Direct Monitoring by Electrospray Ionization Mass Spectrometry. Journal of the Brazilian Chemical Society, 21, 787-794. View Article
Watson, J. T., Sparkman, O. D. (2007). Introduction. In: Introduction to Mass Spectrometry: Instrumentation, Applications, and Strategies for Data Interpretation, Part 4th: Wiley, 3-44 View Article