Volume 1 - Year 2012 - Pages 51-58
DOI: 10.11159/ijepr.2012.008
Treatment Efficiency of an Anaerobic Baffled Reactor Treating Low Biodegradable and Complex Particulate Wastewater (blackwater) in an ABR Membrane Bioreactor Unit (MBR-ABR)
Joseph K. Bwapwa
Mangosuthu University of Technology
Engineering Faculty, Department of Civil Engineering,
P.O Box 12363, Jacobs 4026, Umlazi, Durban, South Africa,
josephkapuku@gmail.com
Abstract - The provision of water and sanitation to poor communities by 2016 is one of the United Nations targets for this millennium. In South Africa many communities aspire to waterborne sanitation. However, there is a technology gap for decentralized and sustainable waterborne treatment plants capable of treating domestic wastewater. Domestic wastewater is more commonly treated using aerobic processes, anaerobic processes may be more appropriate for decentralized applications since they do not require aeration. The anaerobic baffled reactor (ABR) was investigated by analysing physico-chemical and biochemical data from experiments on a laboratory-scale ABR.A pit latrine sludge diluted with tap water was used to feed the ABR. This feed had different biodegradability characteristics compared to domestic wastewater. COD removal ranged from 52 to 80 % depending on the inlet COD. Some COD removal was due to solids retention in compartments, while it was estimated that only 28% of COD removal was due to biological degradation. Soluble extrapolymeric substances (EPS) which are usually a by -product of anaerobic degradation were higher in the feed than in the effluent despite the increasing organic loading rates.More than 50 % of soluble extrapolymeric substances from the load remained in the effluent and were the major cause of membrane fouling for membranes. Also, up to 80% removal efficiencies in terms of total COD and solids were recorded with increasing organic loading rates. While these results do not allow the prediction of ABR-MBR performance during the treatment of real wastewater, it was concluded that most solids retention occurred in the feed tank, COD removal occurred as a result of solids retention and digestion, the loading characteristics influenced only COD and conductivity. The relatively low biodegradability of the feedstock indicates that anaerobic digestion is not the most appropriate treatment for VIP sludge.
Keywords: ABR, OLR, COD, pH, EPS, VIP Sludge
© Copyright 2012 Authors - This is an Open Access article published under the Creative Commons Attribution License terms. Unrestricted use, distribution, and reproduction in any medium are permitted, provided the original work is properly cited.
1. Introduction
1.1. Sanitation Situation in South Africa
One of the United Nations millennium development goals relates to the provision of water and sanitation to previously unserved communities by 2016. In South Africa, despite the fact that significant progresses have been achieved, an estimated 27% of households do not have access to basic sanitation and 16000 people die every year from diarrheal diseases, directly linked to lack of clean water and proper sanitation, 51 schools have no basic sanitation (WRC, 2009). One of the major problems the municipalities face is the delivery of water and sanitation services to those areas located outside the sewered system, mostly in the informal settlements areas where there is lack of formal housing arrangements. These communities sanitation needs cannot be addressed by a centralized approach to sanitation due to time constraints and the large infrastructural investment associated with the technology. Consequently, municipalities have adopted a decentralized approach to sanitation implementing on-site dry sanitation systems. These systems require no water and are less expensive and simple to implement than conventional sewage systems. However, there are many communities which still aspire to waterborne sanitation; as a result, there is a technology gap for decentralized waterborne sanitation systems that are sustainable for these areas. Various technologies have emerged for the achievement of this vision. Even so, the inherent complexity and the high costs are the limiting factors of those technologies in terms of their practical use on a large scale. The ABR was identified by the South African Water Research Commission as one of the ways to address this issue of waterborne sanitation.
1.2 ABR as Decentralized Approach Option
The anaerobic baffled reactor is a high rate digester comprising a series of alternating and standingbaffles in each compartment. Within this type of reactor, wastewater is forced to flow under and over the baffles from one compartment to the next as it passes from the inlet to the outlet (Dama et al., 2002). Bacteria within the reactor gently rise and settle due to flow characteristics and gas production but move down the reactor at slow rate (Barber et al., 1999). This reactor was identified by the South African Water Research Commission (WRC) and the eThekwini municipality as an appropriate sanitation technology for certain geographical areas especially in informal settlements. Its design advantages in treating soluble industrial wastewater have been well documented (Polprasert et al., 1992). In addition, the system has the ability to reduce biomass washout and to separate the spatial arrangement of anaerobic microbial consortia, which confers greater protection from changes in environmental parameters such pH and temperature (Bell, 2002). The versatility and the ability of the ABR in removing organic material, as demonstrated in laboratory-scale projects, suggested its application in the treatment of various wastewaters, including domestic wastewater. Also, ABR is a low cost treatment technology compared to aerobic systems and does not require external energy (Lettinga et al., 1997). Furthermore, it has been proposed that there is a potential to reuse the effluent generated by ABR for horticulture purposes as no nutrient reduction occurs by anaerobic digestion.
2. Materials and Methods
2.1 Laboratory-Scale ABR-MBR
The ABR was designed according to recommendations from a previous pilot study (Foxon et., al 2005) and consisted of a large feed tank (220 l feed capacity) and four identical reactor box compartments (20 l each) connected in series as presented in Figure 1. Each reactor box represents a compartment of the ABR and consists of three identical internal downflow and upflow pipes representing hanging and standing baffles respectively. This modification allows for compartments to be added or removed from original ABR design. Water-seal lids keep the reactor compartments anaerobic and allow for the collection of biogas via a valve connected to plastic Tedlar® bags. All compartments were made of stainless steel (Laser CNC). Each compartment was seeded to one-third of its working volume with sludge from a pilot ABR and the remaining volume filled with a combination of diluted VIP waste and greywater.
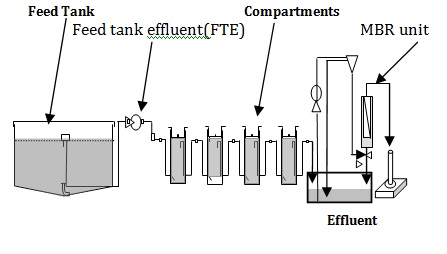
2.2 Description and Preparation of Raw Wastewater
The raw wastewater was comprised of VIP waste collected from VIP toilets in the Magwaveni area near Tongaat (approximately 37 km north of Durban) and Marianhill township. The waste was removed from a single VIP toilet that contained no pit additives and was previously desludged (100 l from top of waste heap) 5 months earlier. The waste was sealed in airtight containers on-site and transported to the laboratory where it was screened to remove undesirable materials such as glass, stones and plastics, and stored in the cold room at 4 °C. A portion of the screened waste (about 1.2 kg of VIP sludge) was removed before daily feeding, diluted with water and homogenised in a blender for 5 min (to prevent clogging). This homogenised waste was further diluted to form an inlet COD concentration between 900 and 3 000 mg/l, which was fed through a lid on the feed tank.
2.3 Objectivesand Experimental Procedure
The main objective chosen to investigate the reactor performance was to understand how the organic loading rates affect the effluent characteristics: to characterize the type of effluent produced by the ABR with increasing loading rates for a reactor treating wastewater from VIP sludge.Therefore, to achieve this goal analyses were performed from the inlet to the outlet of the laboratory-scale ABR. Sampling was completed daily from the inlet (middle of the feed tank), the outlet (effluent collection tank) of the reactor and from the splitter box (Feed tank effluent: FTE).Feed samples were withdrawn after feeding the reactor before starting the peristaltic pump. Effluent samples were withdrawn at any particular time related to the FTE sample from 20 hours previously and the feed characteristics of the previous batch.
The reactor was stopped before samples were withdrawn from each compartment. Supernatant (from the top of each compartment) and sludge samples (from the bottom of each compartment) were collected from each compartment periodically. Supernatant samples were clear liquid with floating solids and sludge samples were very dark and consisted essentially of solids. Polyethylene bottles (l l bottles) were used for sample collection. Samples were kept in the cold room at 4oC with the remaining effluent or wastewater. The experimental approach was based on developing data from physico-chemical analyses during the operation of the reactor. Analyses such as chemical oxygen demand (COD), Total solids(TS), Volatilesolids, pH, temperature, alkalinity, conductivity and extrapolymeric substances (EPS)were conducted according to standard methods (APHA, 1988) on each sample collected from various sampling points at a known frequency asmentioned in table 1. The experimental plan was structured in 5 runs with run 1 known as a test period. During this period the reactor was fed at different loading rates, hydraulic retention times (HRT, average HRT: 3.4 days) and inlet CODs with the primary objective of monitoring various loading rates versus effluent quality. The second period with 4 runs (run 2, 3, 4, and 5) was named continuous feed period. Regarding this period, the inlet CODs were at 1000 mg COD/l during the entire run 2, 1500 mg COD/l for the whole run 3, 2000 mg/l for run 4 and 3000 mg COD for run 5, HRT were kept constant (3 days) for each run during this continuous feed period. It can be noticed from inlet CODs that during this period the loading rates were increased after each run to reduce large variances observed during the first period due to high inlet CODs deviations. To get a precise value for inlet CODs, VIP sludge was diluted in the feed tank using tap water.
Table 1. Frequencies of analyses.
Parameters |
Frequency of analyses |
Sampling points |
pH |
Daily |
Inlet, compartments, outlet, FTE |
Temperatures |
Daily |
Inlet, compartments, outlet, FTE |
Conductivity |
Daily |
Inlet, compartments, outlet, FTE |
COD (total) |
Daily |
Inlet, compartments, outlet, FTE |
COD (soluble) |
Once a week |
Outlet |
Total and volatile solids |
Twice a week |
Inlet, compartments, outlet |
Alkalinity |
Daily (run 6 only) |
Inlet and outlet |
EPS |
Twice a week |
Inlet and outlet |
3. Results and Discussions
3.1 COD
The data presented in table 2 indicates there could be a correlation between the organic loading rates (OLR) and COD removals. The higher is the loading rates the lower will be the COD removal. Run 5 recorded the smallest COD removal. The OLR for run 5 was the highest; solids and microorganisms were washed out during the course of this run. Washout was due to solids removal from the reactor; as a result, microorganisms were destroyed, therefore, the biological activity within the system was affected. Solids were increasing in the reactor and washout was taking place. Consequently, a decrease of COD removal efficiency occurred. Figure 2 shows a sudden increase of feed tank effluent (FTE) COD recorded during run 4 from day 208 to day 210 and during run 5 from day 218 to day 243.
Table 2. COD data from run 1 to run 5.
RUN |
Mean COD ± standard deviation |
Confidence interval at 95 % |
COD removal % |
Run 1 |
Inlet:1561±424 Outlet:303±97 |
[1462,1686] [279,321] |
80 |
Run 2 |
Inlet : 1000* Outlet:309±75 |
n.d [279,321] |
69 |
Run 3 |
Inlet : 1500* Outlet: 334±43 |
n.d [309,353] |
78 |
Run 4 |
Inlet : 2000* Outlet: 457±69 |
n.d [419,495] |
77 |
Run 5 |
Inlet : 3000* Outlet:1439±153 |
n.d [1367,1507] |
52 |
n.d: not determined * unknown variance
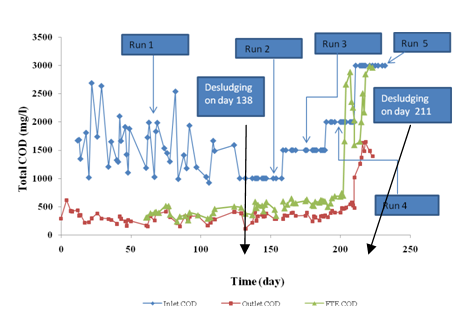
According to the data presented in Figure 2, it was observed that as much as the inlet COD was increasing because of the increasing amount of solids in the reactor, more specially between day 208 and day 223, the FTE COD and the outlet COD have also followed a similar pattern during the same period. Therefore, it can be assumed that the COD load of solids will increase during the period mentioned above and stop accumulating after day 223 because of the overflowing of solids into the reactor (Figure 2).
3.2 General Observations about COD
It can be deduced from the analysis of Figure 2 that the fate of COD (for the feed tank and effluent) depends on the type of COD in such way that inert COD is retained and accumulates within the reactor and eventually it overflows because of the increasing load of solids (wash-through during run 4 and 5) while biodegradable COD is virtually all consumed in the feed tank. Later, the feed tank effluent (FTE) COD started to increase and more biodegradable COD started to enter the system. Also, the fate of COD depends on the conditions and concentrations of microorganisms: the amount of substrate present in the reactor as well as the pH and temperature, may have had an influence on the population of microorganisms and the COD removal. This is an assumption based on the data, however, microbial studies necessary to support this assumption were not part of this study. Therefore, it can be said that the combination of solids retention and anaerobic digestion allowed the removal of organics in the ABR.
COD Mass Balance
The analysis ofTable 3 and Figure 3 indicates that the sludge accounts for 46% of COD consumed in the system with 8% of COD taken by sludge in compartments and 38% taken by sludge in the feed tank. Biogas representing the biodegradability of VIP sludge takes only 28% of COD in the system. Consequently, there is no biodegradable COD in the effluent because it was completely taken by biogas. The biodegradability of VIP sludge (28%) found in this study is close to the one obtained in a parallel study completed by Bakare (2010) which was 28.9%. Nevertheless, biogas data to support the COD mass balance results could not be obtained because the serum bottle method (used to determine Biochemical Methane Potential) was proven to be unsuccessful for VIP sludge (Nwaneri, 2009). However, the analysis of COD mass balance shows that a small fraction of COD is destroyed by digestion (production of biogas) and the remaining big portion of COD was retained by inert solids and biomass present in the reactor. This was happening mostly in the feed tank where large amount of sludge was retained. Therefore, the fact that a small portion of COD is taken by biogas is an indication that biodegradability of VIP sludge is very low.Furthermore, VIP sludge was already fairly well stabilized before being added to the system. This stabilization is due to a permanent biodegradation of VIP sludge that would have occurred in the pit latrine aerobically (top layer) and anaerobically (bottom layer). As a result, there is a low biological activity taking place in the reactor.
Table 3. COD distribution for the ABR operation.
Inflow COD |
46 kg |
100 % |
Accumulated sludge COD(compartments+ feed tank) |
21kg |
46% |
Biogas COD |
13kg |
28% |
Outflow COD |
12 kg |
26% |
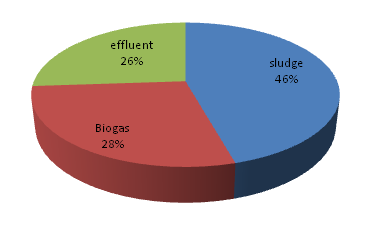
3.3 COD Load of Solids and Estimated Methane Production
COD Load of Solids
Run 5 recorded the highest COD load of solids probably due to high organic loading rate applied during this run. From run 2 to run 6, it was observed that the COD load of solids was increasing from the beginning to the end of each run. This was due to the accumulation of solids from the feed in the reactor, mostly in the feed tank.
Estimated Methane Production
Figure 4 indicates that high production of methane in the feed tank occurred during test period (run 1). During continuous feed period run 5 recorded the highest methane production in the feed tank. This situation is probably due to high OLR applied during run 5. In compartments, the production of methane was not very affected despite the increase in loading rates.
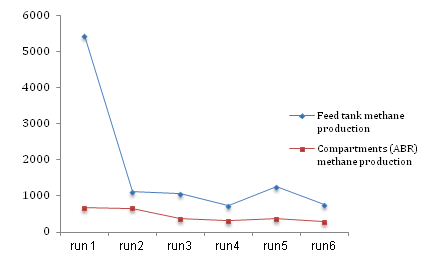
The estimated production of methane in compartments was found to be very low. This was due to the lower biodegradability of the feed material. It can be deduced that this low methane production in the system is an indication that biological stress within the system is ineffective or very low.
3.4 Trend of Soluble and Total COD Concentrations on Effluent Samples
It is observed in Figure 5 that although the slope of soluble COD seems to be changing, the significance of the slope is low. This suggests that soluble COD is not changing substantially with time. Since organic overload and related biological stress is usually observed by an increase in soluble and volatile components, this result indicates that there is no biological stress and the increased total COD is due to wash-through and washout of poorly or non-biodegradable COD. In conclusion, there is no net change in the behaviour of the reactor in terms of biodegradation since there is no change for soluble COD.
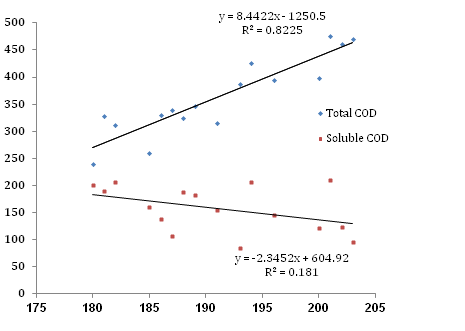
3.5 Reactor pH
During both working periods the effluent pH was between 7 and 7.78 for the effluent except on day 138 when effluent COD was 6.57 and above 7.8 for the feed. Statistical analyses recorded median values between 8 to 8.35 for feed samples (inlet or load) and 7.15 to 7.67 for effluent samples (outlet).The analysis of the data shows a slight decrease of pH observed from the load (inlet) to the effluent (outlet) during both periods. This slight decrease of pH from the inlet to the outlet is an indication of a low alkalinity production in the system. Therefore, low biological activity within in the system coupled with a weak buffering due low alkalinity was the main cause of the slight pH decrease during the treatment process. However, the decrease of pH was not significant and also not harmful for the process because it is suspected that the VIP sludge has low biodegradability which is equal to low acid producing potential.Supernatant samples and samples from the bottom of each compartment were also analysed four times during the operation regarding the pH, it was noticed that there are minor pH variations between compartments. The same observation was made on samples taken from the bottom of each compartment. However, samples from the bottom of compartments had low pH values compared to the supernatant samples. This suggests that a small amount of biological activity was occurring in the sludge bed. Also, it was observed that the feed and effluent pH values were higher than those in each compartment. This was due to the high pressure of CO2 inside compartments which reduces the pH.
3.6 Conductivity
The increasing amount of dissolved molecules during the digestion process taking place in the reactor has a direct influence on conductivity values recorded from the load to the effluent. Overall, it was observed a net increase of conductivity from the load to the effluent during the operation. Statistically, there is a significant increase of conductivity between the inlet and the outlet (Student T-test with unequal variances P≤0.0103). The correlation coefficient between the inlet and the outlet conductivities for all runs is 0.64. This implies a strong relationship between the inlet and the outlet conductivities.The more dissolved molecules are generated during digestion, the more conductivity is increasing.Statistical analyses recorded median conductivities ranging from 366 to 671 μs /cm for the load samples and 462 to 871 μs /cm for the effluent samples. All measurements were achieved at 95% confidence interval from run1 to run 5.
3.7 Reactor Alkalinity
The data recorded indicates that alkalinity increases from the feed (inlet) to the effluent (outlet); low alkalinitieshave been observed during the operation. Nevertheless, it does not mean that the process is close to failure. The type of wastewater used for treatment does not generate high alkalinities; it could be probably due to insufficient production of bicarbonate or ammonia cations indispensable for alkalinity formation. The ability to generate more alkalinity may depend on the nature of wastewater being treated and the presence of proteinaceous compounds which through metabolism generate alkalinity. Therefore, low biological activity within the reactor can be the major cause of low alkalinities recorded during this study. This low biological activity has reduced the capacity of the reactor to release more bicarbonate or ammonia cations which is one of the key factors that allow alkalinity production. The more these cations are released from proteinaceous compounds during the digestion, the more alkalinity can be produced. For this study the average alkalinity recorded for feed is 125±20 mg CaCO3/l and for the effluent it is 140±21 mg CaCO3/l, this is almost 15 times lower than the limit of 2000 mg CaCO3/l suggested by Speece (1996) to buffer an anaerobic system at pH 7.
3.8 Solids in the Reactor
Solids concentrations from the load to the effluent have shown that the ABR has the capacity to retain large amount of solids. The removal of total solids (TS) ranged from 43 to 89% whilst for volatile solids (VS) it ranged from 53 to 90% for different runs such as run 1, 2, 4 and 6. From the experimental point of view it was established that settling of solids within the reactor and the load flow rate were the factors affecting the relationship between the feed and the effluent in terms of solids concentrations. Furthermore, the data shows that the difference TSin -TSout is often not very significant, whilst VSin - VSout is relatively significant; therefore, biodegradation is occurring but does not have a big effect on the solids because biodegradation fraction is relatively small.
3.9 Extrapolymeric Substances (Proteins and Carbohydrates): EPS
During run 2, proteins and carbohydrates were measured in effluent samples only. Run 3 recorded 40% and 39% respectively for protein and carbohydrate removals. Run 4 recorded 43% and 10% respectively for protein and carbohydrate removals. During run 5, the recorded protein and carbohydrate removals were 55% and 21% respectively. Overall, statistic analyses show an average concentration of proteins ranging from (47.5± 16.5) mg/l to (78± 0.5) mg/l for the feed and from (21.4±4.2) mg/l to (71.6±25) mg/l for the effluent. While for carbohydrates concentrations are ranging from (2.3±0.3) mg/l to (2.4±0.6) mg/l for the feed while the average effluent concentrations are between (1.1±0.5) mg/l and (1.9±0.3) mg/l. The analysis of the recorded data indicates that despite the increasing loading rates from run 2 to run 5, effluent EPS concentrations are smaller than feed EPS concentrations. However, the effluent EPS concentrations were expected to be greater than the feed EPS concentrations because of the increasing OLR applied from one run to another. This situation was an unexpected one because it is contrary to what occurs to EPS during any anaerobic process. The understanding of this unexpected situation suggests that the biodegradability of the feed material (VIP sludge) was very low and there was not enough biological activity to produce EPS through metabolism. This low biodegradability of the feed used in this study has validated the fact that the feed material was fairly well stabilized as mentioned previously. Furthermore, despite the fact that there was removal of EPS from the influent to the effluent, it was evaluated from the data recorded for EPS concentrations that more than 60% of EPS remained in the effluent, membrane fouling was observed with rapid decrease in effluent flux from the membrane post-treatment unit (Pillay et al., 2009). Therefore, the EPS present in the effluent were the major cause of membrane fouling in ABR treating wastewater from VIP sludge (Pillay et al., 2009).
4. Conclusion
A four - compartment laboratory ABR treating complex particulate wastewater made up of sludge from ventilated improved pit latrine toilets (known as VIP sludge) was investigated for a period of 264 days. The main focus of this study was to understand the effect of the increasing loading rates on the effluent characteristics; this will define the ability of the ABR to treat complex particulate wastewater. The reactor operated from run 1 to run 6, under a constant hydraulic retention time (HRT) of 3 days at room temperatures. The organic loading rates were increased from one run to another. With the inlet COD ranging from 1000 to 3000 mg COD/l, the ABR produced an effluent with an average COD ranging from 303 to 1439 mg COD/l. This equated to COD removal efficiencies between 52 and 80% for the system including the feed tank and the compartments. COD removal was achieved through solids retention and digestion mostly in the feed tank. The reactor retained large amount of solids during the operation with more than 80 % of solids removal efficiency. Anh et al., (2003 and 2007) reported similar removal efficiencies (for COD and solids) in ABR reactors treating similar type of wastewater in Vietnam. The generated average alkalinity was very low; the type of treated wastewater did not generate high alkalinities. This was probably due to insufficient production of bicarbonate or ammonia cations indispensable for alkalinity production. This low alkalinity generated by the system caused a slight drop of pH from the load to the effluent ranging between 8.9 and 7. Due to the production of dissolved ionic substances during digestion, the recorded effluent conductivities were higher than the feed conductivities. Therefore, with effluent COD recorded from run 1 to run 4 being below the standard for irrigation (400 mg COD/l and a pH above 7), this effluent can serve for irrigation once pathogens are removed by the membrane system in the ABR. However, despite the increasing organic loading rates, it was recorded that most COD was retained by the system through solids retention and a smaller amount of COD was destroyed (through digestion), consequently, less biogas was produced. This is supported and validated by COD mass balance obtained by calculation: 46 % of COD was retained within the sludge while 24 % was taken by effluent, and only 28 % of COD was lost as biogas (which represents the biodegradability of the VIP sludge): it suggests a low biodegradability of VIP sludge. This low biodegradability is an indication that the VIP sludge was already fairly well stabilized before the feeding of the reactor. Furthermore, extrapolymeric substances (EPS) were found to be the major cause of membranes fouling in a parallel project. The data showed that less EPS were recorded in the effluent than in the feed despite the increasing loading rates. Normally, effluent EPS were expected to be higher than feed EPS. It implies that the system was unable to produce EPS. Therefore, this was an indication that low biological activity was taking place in the reactor. These outcomes suggest that large amount of solids settled in the feed tank. Consequently, clogging and solids washout occurred during the operation. Desludging was the only option applied to avoid clogging and solids washout. Mixing was not part of the design to avoid energy consumption. This led to the conclusion that the equipment design did not fit completely the experiment design. It is therefore recommended to use blackwater from waterborne sewage instead of VIP sludge as a feed. This approach will provide more knowledge on the ability of the technology to treat blackwater from waterborne sewage and the effluent type produced by the ABR under known operating conditions. Overall, the ABR could potentially be used as a treatment option in low income communities for the pre-treatment of sewage with higher solids retention and COD removal efficiencies as it was recorded in this study and evidenced in other studies [Foxon et al., (2005) and Anh et al., (2003 and 2007)]. Also, this work has provided an independent assessment of an overall biodegradability of VIP sludge which is of use for proposing sludge management strategies. In this regard, anaerobic digestion is not the most appropriate method for treating VIP sludge however it is suitable as a pre-treatment option.
Future Work
Based on these conclusions, it is recommended to use blackwater from waterborne sewage on the same experimental set up. This approach will provide more knowledge on the ability of the technology to treat blackwater from waterborne sewage. Furthermore, information will be provided regarding the quality or type of effluent produced by the ABR under known operating conditions. Also, with the gain of knowledge on the laboratory scale, implementing pilot plants in the areas in need will be indispensable to test the ability of the technology on site and solve issues of water and sanitation in low income communities.
References
Anh, N.V, Ha, T.D, Nhue, T.H, Heinss, U., Morel, A., Moura, M. and Schertenleib, R., (2003). Decentralized wastewatertreatment – New conceptsand technologies for Vietnamese conditions. In: Proceedings of the 2nd International Symposium on Ecological Sanitation,Luebeck, Germany. pp. 531-534 View Article
Anh, N.V, Nga, P.T, Thang, H.H, Morel, A., and Tonderski, K., (2007). Proceedings of the 16th National Onsite Wastewater Recycling Association Technical Education Conference and Exposition, U.S.A, Paper XI-RCS-07-30
APHA (American Public Health Association) (1998), AWWA (American Water Works Association) and WEF (Water Environment Federation), "Standard Methods for the Examination of Water and Wastewater", pub. United Book Press, U.S.A (ed. by L.S. Clesceri, A.E. Greenberg and A.D. Eaton)
Bakare, T. (2010) Scientific and Management Support for ventilated Improved pit latrine sludge content. PhD thesis, School of chemical Engineering, University of KwaZulu- Natal
Barber, W.P and Stuckey, D.C, (1999). The use of the anaerobic baffled reactor (ABR) for wastewater treatment: a review. Water Research, volume 33 (7), pp. 1559–1578 View Article
Bell, J.,(2002) , "Treatment of dye wastewaters in the anaerobic baffled reactor and characterisation of the associated microbial populations, Ph.D thesis, School of Chemical Engineering, University of Natal, South Africa, p. 1.2View Article
Dama, P., Bell, J., Foxon, K. M, Brouckaert, C. J, Huang, T., Buckley, C. A, Naidoo, V. and Stuckey, D.C, (2002). Pilot-scale study of an anaerobic baffled reactor for the treatment of domestic wastewater. Water Science & Technology, 46(9), pp. 263-270. View Article
Foxon, K.M, Buckley, C. A., Brouckaert, C.J ., Dama, P., Mtembu, Z., Rodda, N., Smith, M.T, Pillay, S., Arjun, N., Lalbahadur, T., andBux, F., (2005). Evaluation of the Anaerobic Baffled Reactor for Dense Peri-Urban Settlements. WRC Report K 1248/1/06, pp. 1–224 View Article
Lettinga, G., Field, J., Van Lier, J.B., Zeeman, G., and Hulshoff, L.W, (1997). Advanced anaerobic wastewater treatment in the near future. Water Science and Technology, 35(10), pp. 5-12 View Article
Nwaneri, C.F, (2009). Physico-chemical characteristics and biodegradability of content of ventilated improved pit latrine (VIPs) in eThekwini municipality. MSc dissertation, School of Biological and Conservation Science, University of KwaZulu-Natal.View Article
Pillay, S., Pollet, S., Foxon, K.M, Buckley, C.A, (2009). Support to the EUROMBRA project: Development of an anaerobic membrane-bioreactor, final report to the Water Research Commission (to be published), Pollution Research Group, School of Chemical Engineering, University of KwaZulu-Natal.
Polprasert, C., Kemmadamrong, P., and Tran, F.T, (1992). Anaerobic Baffle Reactor (ABR) process for treating a slaughterhouse wastewater. Environmental Technology, 13, pp. 857-865 View Article
Speece, R.E, (1996). Anaerobic Biotechnology for Industrial Wastewater. Archea Press, Nashville, Tennessee. View Article